Despite remarkable advances by fiber and disc lasers, the venerable CO2 laser remains competitive in today’s industrial environment.
Jochen Deile and Jim Mozdzierz, Trumpf Inc.
The success of any laser in industrial applications depends on how well it can produce economically positive results for its owner. Despite recent innovations such as disc and fiber lasers, the CO2 laser — which has been around for nearly half a century — still produces very strong economic results, in many cases superior to those of the more recent arrivals. The factors that contribute to the economic success of any laser indicate that CO2 lasers likely will continue as strong competitors for a long time.
From the owner’s perspective, a $500,000 laser cutting machine isn’t a complex piece of sophisticated photonics engineering; it’s a machine that performs the relatively simple task of cutting metal in a manner that maximizes the economic return. To do this successfully, the machine must be reliable, easy to use and versatile. In addition, it is important that the manufacturer offer service, support and training.
For reliability, the system should rarely, if ever, be down for repair or maintenance, and it should be able to run unattended with minimum operator intervention. It should provide consistent performance from day to day with minimum sensitivity to changes in the environment. Also, customers with multiple machines expect consistency from one machine to the next. This can be assured only through products manufactured in consistent assembly lines.
To be versatile, the system should perform well on many different materials with a wide range of thicknesses. It should be able to perform a variety of processes to yield a range of results. All this must be possible with limited or no modification to the machine.
The CO2 laser has dominated the materials processing market because it can meet these requirements. Trumpf alone has more than 15,000 high-power CO2 lasers installed worldwide and is adding almost 2000 units per year. Most of these are used for cutting and are integrated into two-dimensional flatbed cutting machines. Overall, cutting comprises approximately 25 percent of all industrial laser applications, which total about US $6 billion annually worldwide.
CO2 lasers have been successful because they are versatile: They can process almost any material of almost any thickness. A typical cutting machine with a CO2 laser can process material 25 mm thick or more if the system can handle the weight of the material. The lasers can produce the desired results easily if simple adjustments to process parameters are made.
Historically, CO2 lasers could produce more power, with higher beam quality and at lower costs, than other lasers. Multikilowatt CO2 lasers have been available since the early 1980s. Their high beam quality permitted simple beam-delivery systems that reduced the overall cost of cutting machines. Probably even more importantly, their beam quality led to simple focusing heads that could generate the wide variety of intensities needed for diverse cutting processes, without modifying the overall system.
The CO2 laser has been provenreliable; in even the toughest environments, the devices operate very dependably without extensive maintenance. Technological and manufacturing improvements over the past 20 years have led to statistical lifetimes of hundreds and sometimes even thousands of years for the major components of the laser. Even when components need replacement, the substitution often can be done simply and quickly by the owner in his own facility.
Higher-quality cuts
Another parameter that favors the CO2 laser is its 10.6-μm wavelength. Not only is this mid-infrared wavelength eye-safe (i.e., not transmitted through the cornea of the human eyeball), it also has an advantage over shorter wavelengths for cutting because of the subtleties of absorption physics. As a result, the kerf created by a CO2 laser has steeper edges, and the cut is of generally higher quality.
Just as important is the cost of the laser. CO2 lasers often are significantly less expensive than disc or fiber lasers, and their cost of operation is very competitive.
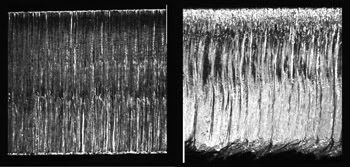
Figure 1. A CO2 laser (left) and a YAG laser (right) were used to make a cut in 10-mm-thick stainless steel. Note the increased roughness of the cut made with the YAG laser.
At the end of the day, the economic return generated by a laser machine tool quantifies its worthiness. Many parameters contribute to maximizing the economic return of a cutting machine. Cutting speed is one parameter but not necessarily the most important one. Often, it takes more time to load and unload a part from the machine or to perform subsequent processing of the part —- welding or bending it — than to perform the actual cutting, so faster cutting is irrelevant in some cases.
There is, of course, a trade-off between speed and quality in that increased cutting speed generally reduces the cut quality, which is defined by the kerf width, the steepness of the cut edge, the surface roughness and the dross. Clearly, it makes no sense to sacrifice quality if the increased cutting speed does not increase overall throughput.
However, other difficulties can reduce reliable cutting speeds and the machine’s economic return, including large variations in the quality of the material and of the assist gas, contamination in the focusing lenses, misaligned beam delivery systems, damaged nozzles and inexperienced operators. The machine must be designed to take all these potential difficulties into account and still provide reliable and mostly unattended operation in the industrial environment.
Cutting processes
Selecting the appropriate cutting process also is an important factor in economic return. The process influences cutting speeds and quality, so a comparison of speeds without taking into account the process employed has little meaning. The CO2 laser can perform sublimation, oxidation, fusion and high-speed cutting.
Sublimation cutting is used for nonmetals. Unlike in the other processes, material is vaporized rather than melted. As a result, no assist gas is required to blow the molten material out of the kerf.
The most common process, oxidation cutting, is used with mild steel. Oxygen is used as the assist gas, and oxidation in the kerf provides additional heat that increases the cutting speed (Figure 2). The oxidation process limits the cutting speed, not the power of the laser. Lasers with more than 4 or 5 kW have minimal advantage, and, in fact, these higher powers could lead to process instabilities. If the finished kerf needs painting after it is cut, the oxidation layer must be removed in a secondary process.
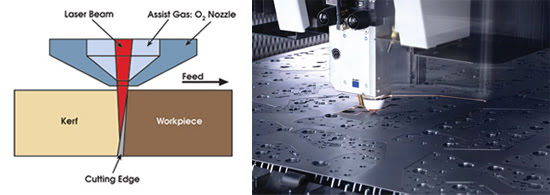
Figure 2. Oxygen is the assist gas in oxidation cutting, and the oxidation reaction provides part of the heat making the cut.In fusion cutting, the assist gas is nonreactive and merely blows the molten material out of the kerf. The picture on the right shows a cutting head, or focusing unit, moving over a workpiece. The cutting head focuses the laser beam and delivers the assist gas.
Fusion cutting uses a nonreactive assist gas, so all the energy comes from the laser itself. (The oxymoronic name of this process has evolved inexplicably over the years.) Mild steel, aluminum, stainless steel and most alloys can be cut with this process. Because there is no oxidation layer, the workpiece can be painted or welded without further processing.
High-speed cutting, also known as laser plasma cutting, is a modified version of fusion cutting. A small pocket of vaporized material forms within the kerf, enhancing absorption. This process requires relatively high intensities, resulting in poor cut quality when compared with fusion cutting. The main difference is the increased roughness of the surface and the possible existence of a slight amount of dross.
Because not every application requires the best possible cut quality, high-speed cutting is appropriate when speed is more important than quality. To reduce the cost further, air can be used as an assist gas. Interestingly, to maintain the vaporized pocket, a certain cutting speed must be maintained. Maintaining this speed requires better drive dynamics and process control, which have become available only in recent years.
All these factors make it difficult to compare directly the economic return yielded by various laser sources. Even a parameter as simple as cutting speed has subtleties that must be considered; otherwise, a laser making slow but high-quality cuts might be deemed inferior to one making low-quality cuts quickly.
Figure 3 compares the reduction in cutting speeds for a CO2 laser using different processes and laser power levels on a 1-mm-thick workpiece of mild steel. Reliable production speeds are only about 20 to 30 percent of the maximum possible cutting speed — the “sever speed” — that the laser can achieve.
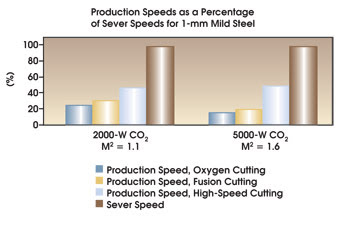
Figure 3. The cutting techniques that can be performed by a CO2 laser require various cutting speeds, but the slower techniques produce higher-quality cuts. The workpiece here was a 1-mm-thick sample of mild steel.
There also is a difference between production speeds and sever speeds for 1-μm lasers. Figure 4 shows the speeds for 2-mm mild steel with three different laser sources. The sever speeds are a factor of two above production speeds. The higher production speeds relative to the sever speeds result from the increased thickness of the material (2-mm mild steel instead of 1-mm mild steel).
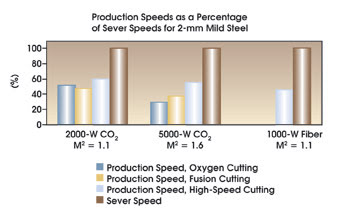
Figure 4. With a 2-mm-thick workpiece, the speed reduction required for high-quality cuts is less than with the 1-mm-thick workpiece in Figure 3. The speed reduction is necessary both with neodymium lasers (λ ~1 μm) and with CO2 lasers (λ ~10 μm).
This dependence on workpiece thickness becomes more dramatic with thicker material, where high production speed and sever speed are almost identical. Figure 5 shows the reductions in speed required with a 12-mm-thick workpiece. In a thick workpiece, the cutting process is dominated by the dynamics of the molten material in the kerf. In thinner workpieces, molten material is disposed of more easily, and beam quality plays a more important role. If a cutting machine will be handling both thick and thin workpieces, a CO2 laser with medium beam quality is the best choice.
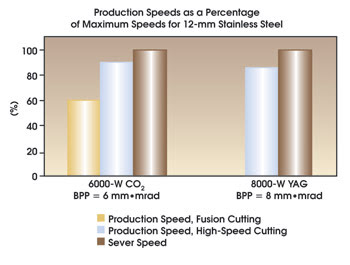
Figure 5. In thick material, the difference between sever speeds and production speeds is reduced because the cutting process is dominated by the dynamics of the molten material and not by the beam characteristics. BPP = beam parameter product.
The CO2 is a mature technology with a long-term, proven reliability, but that does not mean that it will not see future improvements. The source of radio-frequency pump energy, for example, will improve as transistor-based designs reduce cost and cut maintenance requirements while simultaneously improving electrical efficiency and reducing the laser’s footprint. All indications are that the CO2 laser will be with us for a very long time.
Meet the authors
Jochen Deile is the manager of new laser products for Trumpf Inc. in Farmington, Conn.; e-mail: [email protected].
Jim Mozdzierz is an applications engineer in Trumpf’s laser development department in Farmington; e-mail: [email protected].