Research groups are developing carbon nanotubes for imaging and for therapy in biological environments.
David L. Shenkenberg, Associate News Editor
By delivering drugs directly to the source of disease, carbon nanotubes and other nanoparticles could reduce the toxicity that chemotherapy agents and other drugs introduce to the body.
Of all the nanoparticles, carbon nanotubes promise to deliver drugs most safely and effectively. They can carry thousands of drug molecules along their length — many more than spherical nanoparticles can. Thanks to their pure carbon structure, they can be modified with chemical signatures for targeting the source of disease using the vast library of established organic chemistry methods. And because of their long, thin shape, they can slip into cells without causing noticeable damage.
Besides being useful for drug delivery, nanotubes can be heated up by radio and light waves, killing nearby cancer cells. Their electrical conductance has been used for sensing in biology. Finally, their intrinsic near-infrared fluorescence, Raman and photoacoustic properties enable label-free detection and imaging.
Although carbon nanotubes have such great potential, work remains to be done before these applications can become reality. For example, nanotubes require modification with biocompatible materials to eliminate their toxicity. Although they can be modified easily with a range of biocompatible materials, such modification can reduce or eliminate their unique properties.
Past toxicity studies of carbon nanotubes in vivo in animal models mostly have used radio- or fluorescent labels that can decay or detach over time. As a result, the literature has disagreed about the toxicity of the nanotubes. In contrast, the Raman signal is intrinsic to carbon nanotubes, so it will unwaveringly reflect their concentration in the animals. The Raman signal also is strong.
Therefore, researchers in Stanford, Calif., including Hongjie Dai at Stanford University and Xiaoyuan Chen at its school of medicine, have investigated the toxicity of carbon nanotubes in mice using the Raman signal.
“The final goal behind this work is to develop carbon nanotubes as a potential drug delivery vehicle,” Dai said. Toward this goal, the scientists coated the nanotubes with polyethylene glycol, which commonly is used to render substances nontoxic (Figure 2). Chen said that several drugs already approved by the FDA have this coating.

Figure 2. Researchers used single-walled carbon nanotubes coated with polyethylene glycol. They injected them into the tail vein of mice and monitored their in vivo toxicity with Raman spectroscopy. Portion reprinted with permission of PNAS.
In the short term, a drug must circulate in the body to exert a therapeutic effect. But because all drugs carry risks, the drug must leave the body after it has done its job. However, the kidneys often excrete foreign substances such as drugs through urine, and macrophages often destroy such foreign objects outright. The drug would be most effective if it were protected from these mechanisms and eventually excreted through the feces. Therefore, besides studying toxicity of the nanotubes, the researchers also investigated whether various numbers of polyethylene glycol chains and various degrees of branching affected the presence of the nanotubes inside the body. They described their efforts in the Feb. 5, 2008, issue of PNAS.
To perform their investigation, they harvested organs and tissues from mice at one, 30, 60 and 90 days after injection with single-walled carbon nanotubes in solution. They examined these samples with a Renishaw Instruments’ Raman microscope system, collecting the spectra in 10 s each time. They used a 785-nm excitation wavelength because it induces the tangential graphitelike phonon mode, also called the G-band peak area, the strongest Raman signal elicited by the nanotubes. They also mapped the Raman spectra of the nanotubes in the liver using a line-scan Raman microscope, also from Renishaw, at 20 pixels per line in 2 s per measurement.
For nanotubes that had more polyethylene glycol groups with more branching, the researchers saw relatively low immediate uptake by the spleen and liver, which both contain macrophages. The nanotubes also circulated longer in the bloodstream, up to one day, and also more rapidly left the main organs where they were found in about two months. Raman mapping confirmed this effect (Figure 3). The researchers concluded that a greater number of polyethylene glycols with more branching lead to the most desirable effects. “I believe that branched [polyethylene glycols] on other nanomaterials will give you the same effect,” Dai said.
In general, the scientists observed weak G-band signals in the kidney and bladder one day after injection, and the G-band signal in the urine was below the limit of detection. They concluded that only a small number of nanotubes are cleared rapidly by the kidneys.
On the other hand, they found consistently strong G-band signals in the feces as well as signals in the liver and intestine that gradually decreased over the observation period. These results indicated that the nanotubes were excreted primarily through the feces. Dai believes that the excretion of nanoparticles has not been explored to this extent before. “We tracked them for many months,” he said.
The G-band signal was below the limit of detection for organs other than those mentioned. None of the mice died or lost significant body weight. Necropsy, histology and blood chemistry after the three-month period showed no visible signs of toxicity (Figure 3). Therefore, all the results indicated that the carbon nanotubes coated with polyethylene glycol are not toxic.

Figure 3. Raman mapping revealed the distribution of carbon nanotubes in the liver (top row). Histological analysis of liver slices (bottom row) showed no obvious signs of toxicity. Reprinted with permission of PNAS.
Chen said that the FDA requires three years of toxicity data for nanoparticle-based drugs. Dai said that they have already completed another toxicity study, also with the conclusion that the nanotubes are not toxic.
When hit by light, carbon nanotubes make a sound — the photoacoustic or optoacoustic effect. This sound can be detected with an ultrasonic transducer, not unlike the ones used in hospitals around the world to monitor babies in utero.
A photoacoustic detection technique developed by Vladimir P. Zharov and colleagues from the University of Arkansas for Medical Sciences in Little Rock uses both single- and multiwalled carbon nanotubes for real-time detection of single bacterial cells circulating in living mice.
This work also included researchers from the University of Arkansas in Fayetteville and from the Institute of Biochemistry and Physiology of Plants and Microorganisms, and from Saratov State University, both in Saratov, Russia.
Finding single cells
To do this experiment, the researchers exploited the property of carbon nanotubes to preferentially bind to the surface of bacterial cells as compared with binding to the surface of or entering normal somatic cells. Zharov said that it is unknown why bacterial cells have a high binding affinity for nanotubes but that it is “experimental fact.” He believes that the bacterial cell wall may restrict cell entry. Carbon nanotubes also preferentially bind or enter tumor cells, and photoacoustic detection of circulating tumor cells is an active area of investigation, he added.
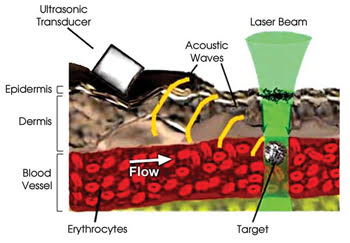
Figure 4. Carbon nanotubes strongly absorb 850-nm laser radiation and consequentially make a sound detectable with an ultrasonic transducer. Because carbon nanotubes strongly adhere to bacterial cell walls and not to a patient’s own cells, the sound indicates the presence of each bacterium in the patient’s bloodstream. Researchers have demonstrated this concept of their photoacoustic technique in living mice. Reprinted with permission of the Journal of Biomedical Optics.
In their experiment, the researchers aimed a laser at blood vessels in the ear or skin of living, anesthetized mice and fired 50-Hz laser pulses for a brief period as cells passed by. In response to the laser pulses, the carbon nanotubes on the bacteria gave off a strong sound. Therefore, the acoustic signal revealed whether or not each cell was a bacterium (Figures 4 and 5).

Figure 5. Researchers performing the photoacoustic technique aimed the laser beam and transducer at blood vessels in the ears or on the skin of the mice. The red color from the fiber is pilot laser radiation.
The researchers call this technique “in vivo photoacoustic flow cytometry” because it counts and classifies cells in the blood vessels similar to conventional ex vivo fluorescence-based flow cytometry, in which cells are fluid-driven through glass capillaries.
However, the conventional method involves examination of a small blood sample of only a few milliliters, so it could miss some circulating bacterial or tumor cells in a patient’s entire 5-l blood volume. All it takes is one of these bacterial cells to multiply and cause sepsis, or one of these tumor cells to metastasize into satellite tumors. In contrast, photoacoustic flow cytometry potentially can detect a single circulating bacterial or tumor cell present in a patient’s entire bloodstream, enabling timely medical intervention that could prevent these life-threatening conditions.
The photoacoustic technique can operate in 10 minutes or less because it can examine blood vessels with a high flow rate, such as the jugular vein. If time is not crucial, it can monitor peripheral vessels in 30 to 45 min.
For in vivo photoacoustic detection, the researchers developed an improved signal-processing algorithm because the previous algorithm provided acquisition times of seconds to minutes, whereas bacterial or cancer cells pass the detected area in milliseconds or faster, Zharov said.
The researchers’ photoacoustic flow cytometry setup was built around an Olympus microscope. They placed an anesthetized mouse on the microscope stage and produced an acoustic signal from the nanotubes by exciting them with an optical parametric oscillator pumped by an Nd:YAG laser — the latter two instruments from Lotis Ltd. of Minsk, Belarus. The oscillator’s wide tunability was useful for the many nanoparticles that were tested in addition to carbon nanotubes. Near-infrared 850-nm excitation was used with the carbon nanotubes.
The stronger the laser pulses, the stronger the acoustic signals, but the nanotubes’ efficiency at converting light into sound enabled the researchers to use laser pulses with power as low as 20 mJ/cm2, well below the ∼34 mJ/cm2 American National Standards Institute safety standard for laser use with humans.
The laser beam’s shape — linear oriented orthogonally to the blood vessel axis — improved the resolution of the measurements, especially for superficial vessels. In deeper vessels, the ultrasonic transducer shape mattered more because scattering dominated.
To detect the acoustic signal, they used a focused cylindrical ultrasound transducer from Olympus NDT of Waltham, Mass., positioned at the ear or on the skin of the live mouse.
The researchers discovered that clusters of nanotubes produced more sound than individual ones. They found that using more single-walled tubes in a cluster was equivalent to using fewer multiwalled tubes. Therefore, they used clusters of both single- and multiwalled tubes. Unmodified nanotubes and DNA-coated nanotubes were used in this experiment, with similar results obtained for both.
Most importantly, the researchers performed real-time detection of single Staphylococcus aureus and E. coli cells circulating in living mice. They observed no toxicity with the carbon nanotubes. This work was detailed in the September/October 2007 issue of the Journal of Biomedical Optics.
Zharov said that they may use this technique in clinical trials with humans this year. For humans, they would use a diode laser array with a high pulse repetition rate, an optical fiber to deliver the laser beam and a readily available diagnostic ultrasonic transducer, both aimed at the skin on the arm. As an extension of this work, his research group, in collaboration with the University of Arkansas in Little Rock, has already combined this technique with in vivo Raman flow cytometry because of the strong intrinsic Raman signal of carbon nanotubes.
From PEG-eggs to pH stability
Researchers at Smalley Institute at Rice University in Houston are developing carbon nanotubes that destroy tumors by delivering chemotherapy drugs and by radiothermal ablation (see sidebar: Hot new therapies). In the process, they discovered a nontoxic coating that improves the brightness and stability of the near-infrared fluorescence signal emitted by the nanotubes.
This work resulted from the collaboration of Howard K. Schmidt and Matteo Pasquali, as well as of Laurent Cognet, who returned to Université Bordeaux I after his sabbatical at Rice.
The researchers used spatially isolated, single-walled nanotubes in their experiment because, as Cognet noted, clustered or multiwalled nanotubes are not prone to fluorescing. Only spatially isolated single-walled nanotubes fluoresce efficiently.
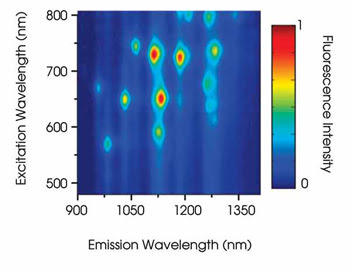
Figure 6. Ensemble spectroscopic measurements of single-walled nanotubes are represented by a rainbow of colors. Reprinted with permission of the Journal of the American Chemical Society.
Nanotubes typically are clustered together as a result of strong attractive forces combined with their straight shape. They usually are spatially isolated with a surfactant, such as soap or detergent, which stays on the nanotubes. However, the surfactant is toxic. Moreover, surfactants are not reliable stabilizing agents in biological environments because biological molecules are known to exchange places with them.
Therefore, such nanotubes need a stable and biocompatible coating to be introduced into biological systems, usually a polymer, which also can assist in drug delivery. For instance, Richard E. Smalley conceived of the “PEG-egg,” with polystyrene on one end to facilitate loading drugs onto the nanotube surface and polyethylene glycol, or “PEG,” on the other end to improve bioavailability of the drug. The PEG-egg was synthesized in 2006 by Paul Engel’s group at Rice. “It works, but it’s hard to make,” Schmidt said.
Previously, researchers had tried another biocompatible polymer, polyvinyl pyrrolidone. However, it made the near-infrared fluorescence signal extremely dim at neutral pH, and most biological compartments are at neutral pH. Furthermore, the fluorescence was not pH-stable.
However, the fluorescence was relatively bright before they added the polymer. Juan G. Duque, a PhD candidate advised by the Rice-Bordeaux team, inferred that the loss of fluorescence resulted from the coiling of the polymer, which disrupted the surfactant conformation, and that this could be avoided by slightly changing the chemistry.
Direct polymerization
In the present experiment, the researchers polymerized vinyl pyrrolidone directly on the surfactant that surrounds the tubes. They tested the nanotubes in solutions of varying pH and ultimately incubated the nanotubes with living cells, as reported in the Feb. 27, 2008, issue of the Journal of the American Chemical Society. “I believe we have used the easiest chemistry that has been proposed,” Pasquali said.
The researchers excited the carbon nanotubes with a circularly polarized 658-nm diode laser. They detected the resulting near-infrared fluorescence with the 60×, 1.4-NA oil-immersion objective of a Nikon inverted epifluorescence microscope, along with long-pass infrared filters from Thorlabs and with a liquid-nitrogen-cooled InGaAs array camera made by Princeton Instruments. “The only thing that’s not standard is the detector,” Cognet said. It’s not easy to make cameras capable of detecting single emitters in the infrared, he added.
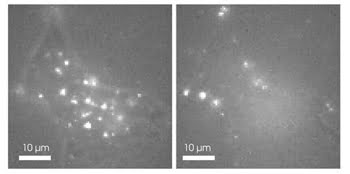
Figure 7. Researchers improved the brightness and stability of nanotubes’ near-infrared fluorescence in cells. Reprinted with permission of the Journal of the American Chemical Society.
The researchers found that the nanotubes’ near-infrared fluorescence remained bright across the entire pH range. “This is the first paper where we show strong fluorescence, even in a highly acidic medium,” Pasquali said, “You keep banging photons on them, and they don’t photobleach.” With living cells, the nanotubes were highly fluorescent and not visibly toxic over the 24-hour period in which they were monitored (Figure 7).
As an extension of this work, Cognet would like to investigate the mechanism of nanotube interaction at the cell membrane. It is believed that nanotubes’ long and thin shape plays a role, but the uptake by cells so far has been mostly taken for granted.
A Tiny Bit of History
Carbon nanotubes can be visualized as tiny cylinders made by rolling up graphene sheets — the basic building block of graphite used in pencils. A ball-and-stick model of graphene’s carbon atoms resembles chicken wire. If you rolled that “chicken wire” into a tube, the resulting structure would be a single-walled carbon nanotube (Figure 1). A multiwalled carbon nanotube has multiple layers of graphene. The number of walls can significantly influence the nanotubes’ physical properties.

Figure 1. Carbon nanotubes have a unique atomic structure that looks like chicken wire. In test tubes, nanotubes look like soot. Portion reprinted with permission of the Journal of the American Chemical Society.
Nanotubes are structurally related to fullerenes — closed-shell molecules comprised entirely of carbon. Their discovery in 1985 at Rice University in Houston culminated in the 1996 Nobel Prize in chemistry. In C60 (buckminsterfullerene), each carbon molecule contains exactly 60 atoms arranged in an icosahedral pattern. The ball-and-stick model of the carbon atoms looks like a soccer ball. Since 1985, many fullerenes have been found, with various numbers of carbon atoms. Therefore, fullerenes comprise an entirely new class of carbon, like diamond and graphite.
Carbon nanotubes were discovered in 1991 by Sumio Ijima and colleagues at NEC Laboratories in Tokyo, and these molecules captured the attention of the late Richard Errett Smalley, who, along with Robert F. Curl Jr. and Harold W. Kroto, won the Nobel Prize for the discovery of fullerenes. Besides studying carbon nanotubes and producing them through his company Carbon Nanotechnologies Inc. (now Unidym Inc.), Smalley vigorously championed nanotechnology funding at the federal level, an effort that helped create the National Nanotechnology Initiative, which has received more than $7 billion and counting during an administration that some have characterized as having an antagonistic attitude toward science.
In an amusing twist, it was discovered that carbon nanotubes can be found among the carbon structures in the ordinary soot that might be found in a fireplace (Figure 1). Nevertheless, carbon nanotubes are far from ordinary. They are stronger than steel, they conduct electricity better than copper, and they conduct heat better than diamond — all in a package 10,000 times thinner than a human hair.
Hot New Therapies
It’s a fact that certain radio signals cause carbon nanotubes to become very hot. Now imagine a scenario in which patients ingest carbon nanotubes prepared with chemical signatures for tumor targeting, and then health professionals use these radio signals to selectively and noninvasively destroy tumor cells.
It sounds like science fiction, but cancer survivor John S. Kanzius invented a radio transmitter for exactly this purpose, and he doesn’t even have a college degree. The device caught the attention of Dr. Steven A. Curley of M.D. Anderson Cancer Center and of researchers at Rice University, both in Houston. In the Oct. 24, 2007, issue of Cancer, the team reported the first radiothermal ablation of cancer cells using the Kanzius transmitter, a first step toward using this technology on patients.
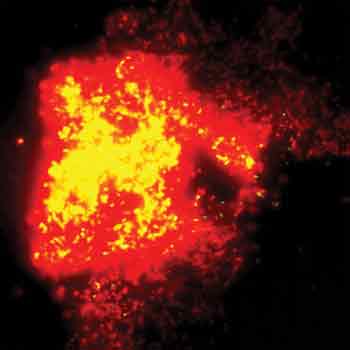
This image looks like a supernova, but it’s actually red and yellow fluorescence indicating the complete death and disintegration, respectively, of E. coli cells bearing carbon nanotubes. Reprinted with permission of Lasers in Surgery and Medicine.
Laser radiation also can cause nanotubes to heat up for treating cancer or bacterial infections. Vladimir Zharov from the University of Arkansas for Medical Sciences in Little Rock and colleagues from the University of Arkansas in Fayetteville have developed a photothermal technique and used it to destroy E. coli. They performed this technique with a 12-ns pulsed Nd:YAG laser from Continuum Biomedical of Dublin, Calif. At both 1064 and 532 nm, all the bacteria bearing nanotube clusters died at fluences ranging from 2 to 3 J/cm2 (see figure). The scientists used both unmodified and DNA-coated nanotubes, and the photothermal treatment was most effective with DNA-coated nanotubes.
Adding ethanol to the bacterial solution helped to increase the efficiency of antimicrobial therapy by more than one order of magnitude through its bactericidal effects and through the enhancement of microbubble formation around the nanotubes, as detailed in the August 2007 issue of Lasers in Surgery and Medicine. Zharov believes that the mechanical strain on the bacterial cells induced by the microbubbles helped destroy the bacteria.