Alana Achterkirchen, Rad-icon Imaging
Over the past four decades, neurophysiologists have studied brain structures and activity using extracellular microelectrodes to characterize the response of neurons in vivo. But there are still many structures of interest located deep within the brain that cannot be accessed directly from the skull surface. Each microelectrode can record only a few cells at a time, so populations of cells are typically sampled serially over the course of many sessions. Yet, the three-dimensional locations of recorded cells somehow must be resolved across multiple sessions.
Additional complexity arises in 3-D brain mapping because the position of a microelectrode can be known accurately only before it is inserted into the brain. The scientist is blind to the electrode’s trajectory and must rely on extrapolation, limiting fine-grained microelectrode mapping to superficial brain structures near the skull surface.
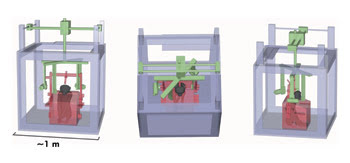
Shown are three views of the imaging system with x-ray cameras and sources in green, the primate chair/carrier in red and the system frame in gray. Courtesy of Cox et al, “High-resolution three-dimensional microelectrode brain mapping using stereo microfocal x-ray imaging,” Journal of Neurophysiology.
For example, small alignment errors at the electrode insertion point can produce large position errors at the depth – at 40 mm, typical for recordings from ventral inferior temporal cortex of nonhuman primates, a 2° angular error at the surface will produce a 1-mm displacement in the target brain structure.
Such effects are compounded by the desire to use thinner microelectrodes to minimize tissue damage, which are inherently more flexible and, thus, less guaranteed to travel straight. As a result, scientists cannot accurately do a “dead reckoning” of electrode target position from the insertion geometry, thus limiting our knowledge of the fine-scale organization of deep brain structures.
Researchers at the DiCarlo Lab, part of the McGovern Institute for Brain Research, Department of Brain and Cognitive Sciences, at MIT in Cambridge, began working with Rad-icon Imaging, a division of Dalsa Corp., in 2003 as they sought to build a digital stereo microfocal x-ray imaging system. Their goal was to produce highly accurate, rapid, real-time estimates of microelectrode position in their brain activity mapping research.
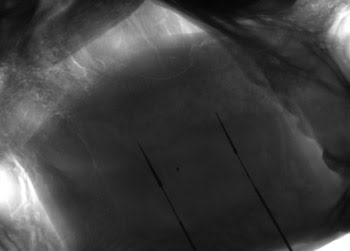
At the DiCarlo Lab at MIT, researchers tested their concept for a digital x-ray imaging system by recording cat skull images with metal electrode markers. Courtesy of Rad-icon Imaging.
The research seeks to advance the neurophysiology gold standard method for localizing microelectrode recording sites, which uses electrical current to produce brain tissue damage at the tip site. The method has severe limitations: It damages the tissue of interest, is very labor-intensive and has limited accuracy, especially in 3-D. Most critically, it requires postmortem observation of the tissue under study.
More recent research leveraged film-based x-ray systems for in vivo studies but faced several limitations. Images were taken serially, requiring greater operator intervention, decreasing the accuracy if the subject moved slightly between image acquisitions and prohibiting any systematic, quantitative analysis of typical accuracy.
Digital x-ray imaging systems are filmless, which makes operation costs of the system negligible. Equally important, they also represent an appropriate choice of technology for use in brain activity research because microelectrode materials are much more opaque than biological tissue. Overall, the digital x-ray cameras from Rad-icon would help DiCarlo researchers build a digital stereo microfocal x-ray imaging system that could quickly and accurately estimate probe locations for in vivo research sessions.
To test their concept for the digital x-ray imaging system, DiCarlo researchers prepared a cat-skull phantom that had metal electrodes and markers embedded in its tissues. Rad-icon’s technical staff tested several x-ray sources together with the phantom and a Rad-icon Shad-o-Box CMOS-based x-ray camera and determined that an Oxford Apogee tube with a 30- to 40-μm focal spot would provide sufficient power and resolution to image the thin, fine-tipped electrodes.
The system would operate at 50 kVp and 1 to 2 mA and would provide approximately 2× magnification at a source-detector distance of 25 cm, suitable for the imaging geometry required for mapping the electrode position in 3-D during the in vivo imaging session. Rad-icon’s CMOS x-ray sensor’s high-resolution 48-μm pixel size enabled the researchers to accurately locate the electrode tip to within less than a tenth of a millimeter.
In 2003, the DiCarlo Lab chose Rad-icon’s Shad-o-Snap 1024 EV digital x-ray camera because of the superior performance characteristics of CMOS technology. The camera enables images to be scanned at megahertz pixel rates and allows for fast image transfer times plus real-time imaging at video frame rates. Graduate student researchers at the lab were planning to make a prototype of a digital stereo microfocal x-ray imaging system. They liked the fact that images could be collected quickly and without interrupting ongoing electrophysiology during their neuronal activity mapping sessions.
While constructing the system, the lab contacted Rad-icon again in February 2005 to purchase two more Shad-o-Snap cameras because the researchers enjoyed the performance and ease of programming to its plug-and-play USB interface in the earlier camera. Rad-icon provides a simple software interface to control the camera and acquire images, but the researchers purchased the Shad-o-Snap SDK and, with guidance from Rad-icon, wrote their own C-based software.
The stereo imaging system was completed by the end of 2005 but required three computers to control the two Shad-o-Snap cameras and the overall system. At the time, undergraduate student Jonathan Karr was given the task to simplify the imaging system design so that all functions could be combined in a single PC. This required upgrading the Rad-icon firmware and SDK so that multiple cameras could be attached to the USB hub on the computer.
In March 2006, he contacted Rad-icon tech support with his plan. The company’s technical team devised a way not only to allow multiple Shad-o-Snap cameras to coexist on the same Windows system but also to be able to control them with minimal overhead, using the same SDK functions as before. This required Rad-icon to update the registry identifiers in the camera firmware, but the cameras were reprogrammed and the software updated before the end of the month. Karr was able to greatly simplify the imaging system, resulting in cost savings, reduced complexity and increased operational reliability.
The design specified a pair of x-ray images to be acquired at each microelectrode recording site using two x-ray sources from Oxford Instruments of Scotts Valley, Calif., each projecting its beam onto a 1-megapixel digital x-ray camera from Rad-icon. The approach opens up new potential for high-resolution physiological 3-D mapping of brain activity in awake, behaving animals. In an article published Sept. 24, 2008, in the Journal of Neurophysiology, DiCarlo Lab researchers studied two rhesus macaque monkeys. They performed animal training, surgical procedures, eye monitoring and recording methods using standard techniques for awake primate visual neurophysiology.

A pair of x-ray sources project x-rays through a region containing a recording microelectrode onto a pair of digital x-ray detectors from Rad-icon. Courtesy of Cox et al, “High-resolution three-dimensional microelectrode brain mapping using stereo microfocal x-ray imaging,” Journal of Neurophysiology.
The source-detector pairs were affixed to a custom-built gantry that held the sources and detectors from above. The gantry enabled the apparatus to be lowered, translated and rotated around the animal’s head, with each source-detector pair rotating independently around a common center point. The two source-detector pairs were rotated so that the “spread” angle between them was as close to 90° as possible, ensuring that both pairs had a clear shot of the microelectrode and external fiducial markers and that the source-detector pairs minimally occluded the visual field of the animal. As a result, they acquired x-ray-based microelectrode tip position estimates for several hundred recording sites over the course of a standard electrophysiological experiment.
This new method is practical for many neurophysiology laboratories. It can be deployed on a day-to-day basis and is minimally intrusive to ongoing experiments because images can be acquired in a few seconds while a recording session is in progress.
The lab’s images were acquired simultaneously by both source-detector pairs, with an integration time of 4 s. Under computer control, the sources were electrostatically shuttered, using the x-ray tube’s focus terminal to deflect electrons away from the tube’s anode target, enabling precise on-off control over exposure. The system cost was ~$40,000, not including machining and labor. The x-ray sources were ~$6,000 each and the x-ray detectors, ~$13,000 each.
The technique constructed by the DiCarlo research team substantially extends previous neurophysiology methods because all microelectrode sites recorded on a single day – typically 10 to 20 – can be localized in real time to guide the ongoing experiment. An unlimited number of recording sites can be individually localized and co-registered over many experiments, resulting in unprecedented neurophysiology maps that have both high spatial resolution and a large field of view.
The researchers also demonstrated that mappings can be measured deep within the brain, where the accuracy of traditional microelectrode mapping techniques normally breaks down. The digital x-ray imaging system’s ex vivo localization accuracy is better than 50 μm and can be used to co-register hundreds of deep-brain recordings to a common frame of reference with a median error of 150 μm.
The DiCarlo Lab’s approach will fundamentally yield new kinds of high-resolution maps of brain function. Future researchers will be able to build highly detailed 3-D maps of brain activity by collecting large numbers of spatially resolved microelectrode recordings. These maps have the power to reveal details of cortical microstructure even in deep-brain regions where such structures are currently very difficult or impossible to measure.
Meet the author
Alana Achterkirchen is the director of business development and marketing for Rad-icon Imaging, a Sunnyvale, Calif.-based division of Dalsa Corp.; e-mail: [email protected].
Reference
D.D. Cox et al (September 2008). High-resolution three-dimensional microelectrode brain mapping using stereo microfocal x-ray imaging. Journal of Neurophysiology, pp. 2966-2976.