Astronomy may be the oldest science, but today it’s driving development of the newest instrumentation, coatings and optical components. In part these advances are due to the intersection of astronomy and photonics that’s been dubbed “astrophotonics.” Such innovations, together with the next generation of telescopes, may help answer two fundamental questions: “Are we alone in the universe” and “How did everything start?”
But first, astronomers have to overcome a basic problem: Their research is light-starved.
The solutions to this situation are pushing the state-of-the-art and could prove beneficial for more than stargazing, said astronomy and astrophysics professor Joss Bland-Hawthorn of the University of Sydney in Australia. “There are important overlaps to microscopy, to remote sensing and to medical applications.”
Adding urgency to this momentum in astronomy technology are the large ground-based optical and infrared telescopes scheduled to be built over the next 10 years. These giants will span as much as 42 m, dwarfing existing 10-m telescopes. These and other new telescopes could be able to spot Earth-like planets orbiting distant stars. They also could capture first light, the time in the history of the universe when the first stars began to shine.
Such discoveries will only be possible, though, with a revolution in instrumentation. A look reveals what’s needed and how those demands are being met.
Making bigger work
The next decade, according to current schedules, should see construction of the 24.5-m Giant Magellan Telescope, the Thirty Meter Telescope and the 42-m European Extremely Large Telescope. One of the reasons why such monsters can be built – and do good science – is adaptive optics. This technology adjusts the surface of a small, flexible mirror to cancel out distortions in the atmosphere. As a result, stars don’t twinkle, and the viewing is almost perfect.
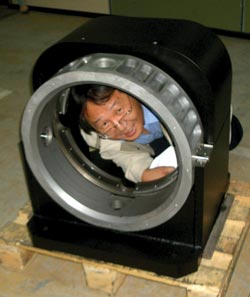
Shown is a prototype adaptive optics demonstration mirror for the Thirty Meter Telescope. Courtesy of CILAS.
Adaptive optics has been successfully used to improve the performance of current 8- and 10-m telescopes. However, because the correction has to be done at a certain speed and over a given distance, a tripling in diameter means there’s nearly a tenfold performance increase demanded of components.
“The detectors have to be bigger. The deformable mirrors have to be bigger. The signal processors have to be able to do computations more rapidly,” said Brent Ellerbroek, group leader of adaptive optics for the Thirty Meter Telescope.
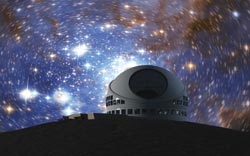
An artist’s rendering shows a time exposure of the Thirty Meter Telescope at night at one of its candidate sites. Courtesy of TMT Observatory Corp.
Lasers are another element that must be improved. In adaptive optics, light from a star is captured and sent through a wavefront analyzer and a mirror that is adjusted to smooth out atmosphere-induced aberrations.
This requires that a bright enough guide star be near an object. Since this isn’t always the case, astronomers make their own, firing lasers at a sodium layer that floats about 100 km, or 60 miles, above the Earth. There’s a desire to tailor the laser output, which should be centered on 589 nm, to get the best possible artificial guide star.
In the case of the Thirty Meter Telescope, the adaptive optics will involve two or more deformable mirrors in a multiconjugate adaptive system. This approach will increase the correctable field of view compared to what would be possible with a simpler system.
The goal is to get the remaining residual wavefront aberrations down to 190 nm root mean square (rms) by the time the telescope collects its first light. Plans are eventually to get this down to 130 nm rms. That’s better than the 300 nm rms typically possible today, and it will require some advances. “We are asking for incremental improvements: a little more stroke, a little closer spacing, operation at somewhat colder temperature,” Ellerbroek said.
He added that a high-stroke, low-temperature demonstration mirror with a 9 x 9 array of actuators has already been built, as well as a prototype tip/tilt stage and various electronics. Thus, the components are on track for the 2018 scheduled completion date of the telescope.
Big scopes, small instruments
While the Thirty Meter Telescope and its brethren are large, paradoxically they will demand devices that are more integrated and miniaturized than those required by their current counterparts. That’s because the new telescopes are expected to collect data from as many as 100,000 objects simultaneously, with the light from each routed individually.
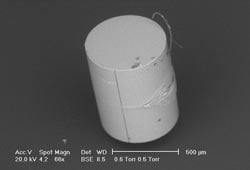
With subpicosecond pulse power ultrafast laser inscription, astronomers hope to carve out complex optical structures. Here the technique has been used to create a 700-μm-diameter, millimeter-long cylinder of fused silica. An acid bath preferentially etched a laser-irradiated region, causing the cylinder to drop out onto a plate. Courtesy of Robert Thomson, Heriot-Watt University.
To get the greatest number of photons through, multimode optical fibers will be used. However, existing devices often work best with single-mode input. Hence there’s a need for a device for the low-loss transition from multi- to single-mode.
Simply scaling up existing instruments isn’t feasible. Neither is churning out thousands of modules using technology. So astronomers are turning to new approaches.
One proposed solution comes from photonics researcher Robert R. Thomson and professor Ajoy K. Kar of Heriot-Watt University in Edinburgh and from astronomer Jeremy Allington-Smith of Durham University, both in the UK. In a February 2009 Optics Express paper, they advocate the use of ultrafast laser inscription, a somewhat new technique in which sub-picosecond pulses focused inside a transparent material alter its properties. The method can change the refractive index of a glass substrate or lower its resistance to chemical etching, enabling fabrication of complex three-dimensional optical structures.
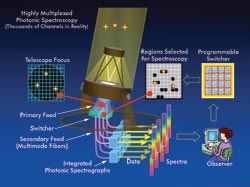
Miniaturized photonic spectrographs will be used for highly multiplexed spectroscopy with next-generation telescopes. Several thousand channels might be available to allow astronomers to address fundamental questions about the evolution of the universe. Building such systems could be done with ultrafast laser inscription. Courtesy of Robert Thomson, Heriot-Watt University.
To date, ultrafast laser inscription has been used only for relatively simple devices. However, Thomson noted that this use has proved the method’s reliability; moreover, he added, the approach has significant advantages. “Although the initial fabrication and optimization of these devices may be challenging, the subsequent fabrication of many devices will be easily achieved by automating the inscription process.”
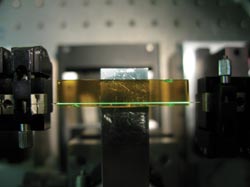
This 40-mm-long erbium-doped waveguide optical amplifier was created using ultrafast laser inscription. Courtesy of Robert Thomson, Heriot-Watt University.
The fabricated devices do suffer from relatively high propagation loss and low refractive index contrast. The first robs astronomers of needed photons, while the second increases device size by limiting minimum waveguide bend radius. The researchers believe, however, that these problems can be overcome and are working to implement fixes.
If they’re successful, Thomson noted, the technique could prove useful in a variety of settings. “The ability to create micro-optics, micromechanics, integrated optics, microfluidics and optofluidics – all on the same chip, all using the same fabrication route and, on top of all of that, in three dimensions is an incredible capability.”
Putting nanodetectors to work
Astrophotonics is also at the heart of another proposed solution for a different problem, that of combining coherent beams for stellar interferometry. This technique allows widely separated telescopes to perform like a single one as large as the spacing between them.
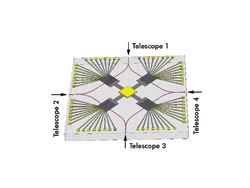
In this beam combiner design for a four-telescope configuration, nanodetectors and integration eliminate the need for a complex and difficult-to-adjust optical setup. Courtesy of Pierre Kern, Laboratory of Astrophysics, Grenoble Observatory. Reprinted from Optics Express.
Pulling this off for many beams without a complex optical setup requires an integrated instrument that includes coherent beam combination, spectrometry and detection. Advances in technology could now make that possible, reported a group of French researchers in the February 2009 Optics Express. The concept works by placing nanodetectors along standing waves in a single-mode waveguide. If the detectors are spaced properly, the device can probe spectral information without having to scan anything.
The researchers outlined three concepts exploiting this method. One is a photo-counting fringe tracker based on superconducting single-photon detectors. Such detectors are under development at labs worldwide, but they’re currently not as well established as the devices used in the other concepts – focal plane arrays based on CCD or CMOS technology.
Research team member Pierre Kern, an engineer with the Laboratory of Astrophysics at Grenoble Observatory in France, said of superconducting single-photon detectors and the group’s concept of how to make use of the technology, “It is an ideal setup, but its implementation will require a longer development time.”
Don’t forget the little guys
Of course, bigger isn’t always better. For instance, one way to detect exosolar planets is to look at the light from a star. When a planet passes in front of a star, the star’s light dims. That’s the idea behind the recently launched NASA Kepler mission, and the technique can be used by ground-based telescopes as well.
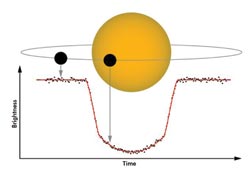
A planet orbiting a distant star causes the star to dim if the planet passes between the star and Earth. Here’s the light curve for Wasp-10, whose planet, Wasp-10b, blocks approximately 2.5 percent of the star’s surface. Courtesy of John Johnson, Institute for Astronomy, University of Hawaii.
Measuring the light curve rapidly and with high precision allows astronomers to spot the planet, as well as to determine its size and orbit. However, finding time to do this on a large telescope can be hard to justify, as it requires spending an entire night on a single target.
There’s a second issue where a bigger telescope can’t offer improvement, said John Johnson, an astronomy postdoctoral fellow at the University of Hawaii in Honolulu. “The problem with stars that harbor planets is that they’re too bright.”
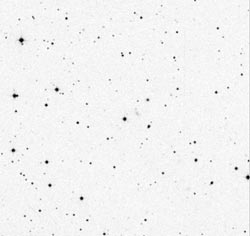
Wasp-10, a star about 300 light-years away, is just a dot in a star field, but astronomers know from dips in its light output that it has a planet. Courtesy of John Johnson, Institute for Astronomy, University of Hawaii.
Consequently, the pixels of a detector will saturate within seconds, making it impossible to pick up a light curve blip. One way around the problem is to defocus the telescope, but that can lead to errors.
Johnson and fellow researchers demonstrated a better solution, one that runs on a 2.2-m telescope and uses a new type of detector: the orthogonal transfer array. It differs from a standard CCD in that it has four gates instead of three, and a channel stop. This difference allows accumulated charge to be shifted in two dimensions instead of just one during an exposure.
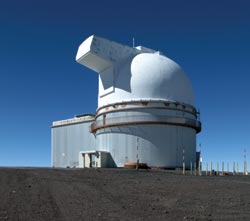
Shown is the University of Hawaii’s 2.2-meter telescope dome on Mauna Kea. A detector that allows more pixels to accumulate photons enabled astronomers to use this telescope to make a more precise measurement of the size of the planet Wasp-10b. Photo by Karen Teramura.
The detector was co-developed by MIT Lincoln Labs and John L. Tonry, an astronomer at the University of Hawaii. Tonry noted that the detector allows tip-tilt correction to be done in the silicon itself and so permits image stabilization. “I have mostly used it for sharpening images which quiver at 10 to 100 Hz from telescope and atmospheric shake,” he said.
Johnson and the group did the opposite, smearing the image and spreading out the accumulated signal over pixels within a well-defined region. They used the technique to measure the light curve of the star Wasp-10, which is about 300 light-years away. They achieved a photometric precision of 475 parts per million, or about one part in 2000. That is three or four times better than had been done previously.
In an Astrophysical Journal Letters paper, the researchers reported that the technique allowed them to determine that a known planet, Wasp-10b, is about a sixth smaller and twice as dense as had been previously thought. That brings the planet in line with theory.
As for the future, Johnson is planning to search for transits of planets with masses between those of Earth and Neptune. Orthogonal transfer array, or OTA, detectors will prove useful for this and other investigations of extrasolar planets, he predicted. “OTAs are truly a killer app[lication] for transiting exoplanet research. Stay tuned over the next year or so.”
Small Satellites, Big Requirements
Astronomy, of course, isn’t done just on Earth. There have been space-borne systems for decades, and this year could see the latest addition, a Canadian cluster of cube-shaped satellites that each measure 20 cm, or 8 in., on a side. Within those confines, the satellites will carry a CMOS detector, imaging optics and everything else needed to do research.
The BRIght Target Explorer, or BRITE, fleet will allow astronomers to photometrically measure low-level oscillations and temperature variations in 286 bright stars. Expectations are that the constellation will enable unprecedented precision and time sampling that are not achievable on the ground.
But first those satellites had to be built, and that proved to be a challenge, said Kirk Winchester, product manager for professional astronomy at filter maker Omega Optical of Brattleboro, Vt. Space-traveling instruments face a harsh environment and often require small and light optical components. In the case of the BRITE mission, there also was a need to achieve better filter performance, with steeper slopes and higher transmission.
To meet these requirements, Winchester said the company applied the latest technology, dual magnetron reactive sputtering. The system uses optical and other means to monitor the physical thickness of layers as they are being deposited, with adjustments made on the fly.
Such capabilities may come in handy for ground-based telescopes as well. The filter sizes, driven by the increase in the detector sizes, are growing. That presents a problem, but one that the new coating technologies may help solve. As Winchester noted, “The filter functions are based on precise layers of high- and low-index materials that have to be a certain thickness. The more you spread that out over an area, the more difficult it is to keep that uniformity.”