Fiber lasers and thin-disk lasers have been the focus of intense development recently because they can efficiently convert the high-divergence output of powerful diode lasers into narrow, high-power beams for countless industrial applications.
Breck Hitz, Senior Technical Editor
What led you to investigate alkalis as laser materials?
I’m an inventor, an applied scientist. I like asking what the problem is and then looking for solutions to it. At any given time, we have an arsenal of lasers and we often try to force them to perform applications they’re by no means ideal for.
The advent of diode pumps transformed solid-state lasers into a whole new class of device that was able to address elegantly a whole new set of applications. But keep in mind, this happened in 1983 — the concept is 20 years old! I’m proud to have been one of the first people to work on that technology. But it seemed to me it was time to revisit the entire issue. Solid-state technology is still struggling to get good beam quality at maybe 2 kW. In the end, it’s all about waste heat and thermal management.
In any diode-pumped solid-state system, the solid-state gain medium is the weak link. Diodes are 50 percent efficient and going to 80 percent, I guess, if DARPA has its way, and they have good potential for low cost and manufacturability. So I started looking for a way to replace the solid-state gain medium with a gas. I wanted to capture the low medium density, the prospect for no thermally induced birefringence, the prospect for convective cooling — you know, the key characteristics that people tout for gas lasers. But now I would have the advantage of pumping the gain medium with a concentrated source of radiation in the form of a laser diode.
And that led you to the alkalis?
Yes, but not immediately. I didn’t start with the alkalis. I spent a year going through the periodic table, column by column and molecule by molecule, trying to find a match between diode characteristics and the desired high-brightness converter —an optical impedance match, if you will, something that could convert one to the other. The problem is, even though diodes are spectrally narrow compared with lamps, they’re still much broader than most atomic or molecular absorptions. How can you capture pump radiation in a useful way in atoms or molecules?
When I got to the alkalis, I thought, “great, they’ve got very strong transitions but they’re incredibly narrow. How can they ever be made to work?” But alkalis have been so thoroughly studied during the past 70 or 80 years that all the parameters are well-known, and it was relatively easy to grind it all up and put it into a computer in a fairly simple model — just an Excel spreadsheet, basically — and come up with some interesting results.
What you absolutely need to have is a way to broaden the absorption line. You don’t have to broaden it as much as the diode’s bandwidth, but just enough so it’s Lorentzian and effectively spectrally homogeneous. That way you can pump the transition in the wings and yet have the absorbed energy contribute to the center of the gain transition. And it turns out that you can do that by collisionally broadening the alkali’s absorption with maybe half an atmosphere of helium.
So were you home free at that point, or were there other issues to solve?
The next thing you have to do is get the pump radiation from the terminal level of the pump transition to the upper laser level, and you need to do that quickly, compared with the level’s ~28-ns spontaneous decay lifetime. But if you buffer the alkali vapor with 100 torr or so of a small molecule like ethane, you get collisional mixing of those two states on a time scale an order of magnitude faster than the spontaneous decay. So the terminal pump level and the upper laser level are essentially in thermal equilibrium.
So we’re talking about a three-level system, right? An alkali laser has its ground state, its pump-to level and its upper laser level?
Actually, I’ve started calling it a quasi-two-level system, because the upper two levels are in thermal equilibrium. But, yes, in the conventional sense, it’s a three-level system. Nonetheless, the overall conversion efficiency can be quite high, in part because the quantum defect is extremely low, about 2 percent for rubidium. Compare that with neodymium’s 24 percent quantum defect, or ytterbium’s 8 percent.
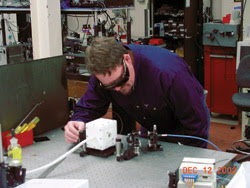
V. Keith Kanz of Lawrence Livermore National Laboratory adjusts a 795-nm rubidium laser.
The high conversion efficiency means we have less waste heat per excited atom to deal with than in a solid-state laser. On the other hand, it’s harder to get the heat out by conduction. A gas, even helium, isn’t that good a thermal conductor. So there’s no free lunch. You get less heat, but it’s harder to deal with that heat. The refractive index in a gas is much less temperature-sensitive. All the numbers are so different than with a solid-state laser. So you get a sort of new multidimensional space to play in, which gives you different degrees of freedom in designing a laser for a given application.
In an era when solid-state lasers are replacing gas lasers, the development of alkali-vapor lasers seems an anachronism. How difficult is it to work with an alkali vapor? Is it dangerous?
No. I mean, they are reactive, but it’s a relative thing. Graduate students have spent 50 years learning how to handle alkalis safely. Rubidium vapor is now used commercially in atomic clocks. They’re guaranteed for many years of operation, and people have developed specialty glasses that don’t react with alkalis.
Having convinced yourself that alkali vapors had the potential for a new approach to the diode-pumped laser, what did you do in the lab?
We actually used the rubidium-vapor cell of a commercial atomic clock, a design based on what we could buy, rather than what we really wanted. We end-pumped it because we knew we needed the long absorption length to increase the opacity of the far wings of the absorption line. We used a Ti:sapphire laser at 780 nm in the initial demonstration because it’s easier to work with, but the intention is eventually to demonstrate diode pumping. We put the rubidium cell in an oven so we could control its temperature at 120 °C to within a degree. The rubidium density was about 1.7 × 1013 cm3.
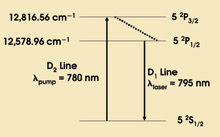
This diagram depicts the energy levels for rubidium.
The laser produced 25 mW at 795 nm, with a slope efficiency of 54 percent. And we were gratified to see how closely our model predicted the experimental behavior we observed. We varied both the output coupling and the cell temperature, and saw the output power following the model’s predictions very closely.
What’s happened more recently in the lab?
We’ve been working with cesium. We did an even more extensive experiment in the sense that we measured everything, all the parameters. We wanted to compare our results with our theory, and we got perfect lockup — incredible. That gives us a lot of confidence in making projections to much higher output power, and with diode pumping.
Do you expect diode-pumping results soon?
We want to explore the range that gets us up to 5 or 10 atmospheres of helium, pressures that accommodate the use of commercially available diode bars with spectral widths of a few nanometers. We need diode lasers at 780 nm, which today have limited brightness. It’s work intensively in progress right now. We could have results today, could have results next week.
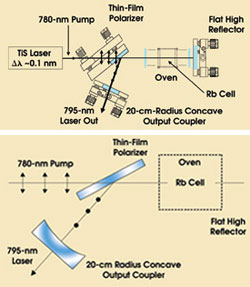
Pictured are an experimental arrangement for (top) and an optical schematic of (bottom) the rubidium laser.
Both alkali lasers and thin-disk lasers are being developed to convert the low-brightness, high-power output of diode lasers into high-brightness, high-power lasers. What are the trade-offs between the two?
It’s probably too soon to give a definitive answer to that question. The thin disks are certainly more advanced. I guess Trumpf introduced a commercial product at the Munich laser show last year.
One difference between the two is that alkali lasers can develop enormous gains — 100 percent per centimeter is easy. So they are well suited for mode control using unstable resonators, which provide means for extracting near-diffraction-limited power from a large-mode volume, with a large aperture. That’s not possible with thin disks, which have a very low gain.
But from an overall point of view, I see thin-disk lasers and alkali lasers as being in the same space. We still have to prove that alkali lasers are capable of high-power operation with the same optical-to-optical efficiency as thin disks. But if we do, then the two are likely to be similar as far as cost and performance are concerned. In either case, the cost is going to be dominated by the diodes. The converter is relatively inexpensive.
What about fiber lasers? They’re also being developed to convert diode-laser outputs to usable beams. How do they fit into the equation?
Well, I’m a fan of fiber lasers. We’re going through a period of enormous innovation in guided-mode structures — large-area mode fibers and so forth. A major invention was the double cladding, which gave them a way to couple pump power into the fiber efficiently. You know, I think fiber lasers are going to set the bar for other high-power, high-brightness lasers to measure up to. But … I think that alkali lasers certainly have the potential of measuring up.