Prized for its rapid 3D imaging capability, low phototoxicity and high contrast, Airy beam light-sheet microscopy is especially well-suited for cancer biology and neuroscience.
KISHAN DHOLAKIA and JONATHAN NYLK, UNIVERSITY OF ST. ANDREWS; PETE PITRONE, GRAEME MALCOLM and ROBERT FORSTER, M SQUARED LASERS LTD.
Airy light-sheet microscopy (ALSM) has great potential to help further research and clinical diagnoses through its ability to image larger 3D volumes of tissues and organoids. Developed in the last three years, it is best-suited to the fields of regenerative medicine, cancer biology, neuroscience and developmental biology, as ALSM can acquire images, as well as 3D datasets, with higher resolution over larger fields of view (FOV) than its predecessors.
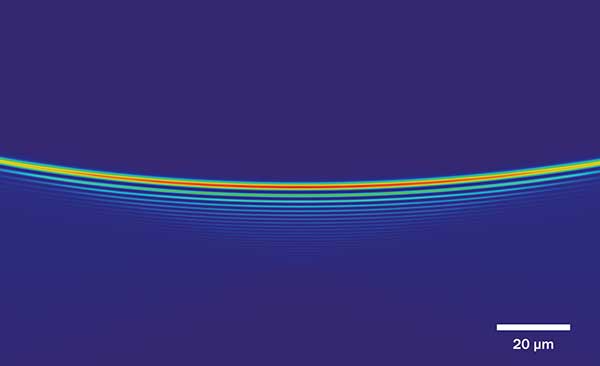
Profile: Airy beam parked in the focal plane of the detection lens. Courtesy of University of St. Andrews, School of Physics and Astronomy/Kishan Dholakia, Jonathan Nylk.
One such predecessor is fluorescence microscopy, a powerful tool that life scientists rely on to observe biology in action. It works by using a light source that produces excitation photons of a certain energy, one of which then excites a fluorescent molecule, called a fluorophore. The fluorophore attaches to a specific location on the specimen, causing it to fluoresce, emitting a photon of a lower energy.
For example, if the fluorophore excites when exposed to blue light it would most likely emit in green, yellow, orange, or even red. For acquiring fluorescence images, one needs to block the excitation light from the detector, while collecting as much of the emission light as possible.
There are two main issues with fluorescence microscopy: phototoxicity, which damages the specimen, and limited penetration into the sample, which results in the loss of information both inbound and outbound. High concentrations of energy have phototoxic effects: High energy wavelengths in the blue-violet UV part of the spectrum cause damage to biological material, whereas bleaching, resulting from the continued toggling on/off of the excitation of the fluorophores, causes the emergence of free radicals. This can sometimes make in vivo study of fluorescent specimens challenging as cells die more quickly.

A diagram of the beam path of a conventional fluorescence microscope, along with its illumination pattern (top right). Courtesy of Mllyjn – Own work, CC BY-SA 3.
Light-sheet fluorescence microscopy
Light-sheet fluorescence microscopy (LSFM) is attractive because it increases the speed of data collection, and its deep penetration allows for optimal fluorescent excitation of yet larger sample areas with depth selectivity. LSFM subjects the specimen to reduced phototoxicity, which is important for sampling specimens in vivo in developmental research, and captures images at a much faster rate than point scanning confocal microscopes.
In conventional fluorescence microscopy the excitation light travels along the same path through the microscope objective that the emission light comes back on. Due to the fact that the excitation light is always so much more intense than emission light is, and the way it is directed into the sample, a 3D hourglass shape is excited in front of the objective with the focus being the smallest point. LSFM decouples the illumination path from the detection path by 90 degrees and illuminates only the focal plane of the detection lens with a “2D” sheet of light. This is achieved in one of two ways: by creating a static light sheet with cylindrical lens optics, or by rapidly scanning a beam across the field in a direction perpendicular to both the illumination and detection axes.

Profile: Gaussian beam parked in the focal plane of the detection lens. Courtesy of University of St. Andrews, School of Physics and Astronomy/Kishan Dholakia, Jonathan Nylk.
Superresolution
Some of the challenges in applying microscopy to scientific research, industrial applications and other contexts go well beyond just acquiring images at a high magnification. One of these challenges is resolution. With visible light, the diffraction limit presents obstacles to imaging at a resolution any finer than 200 nm. One can resolve smaller structures by using superresolution microscopy techniques.
There are two approaches. The first technique introduces known patterns to the back focal plane of the microscope objective to tease out details that allow researchers to resolve distinctions at a distance of 100 to 120 nm apart (a factor of 2× better than the diffraction limit). This requires multiple images of the same plane, so it takes much longer to acquire.
The second method involves localizing individual fluorescent markers by randomly toggling them on and off, using a technique called stochastic optical reconstruction microscopy (STORM). In contrast, photo-activated localization microscopy (PALM) utilizes fluorophores that can be photo-activated to change their characteristics, allowing researchers to resolve distinct objects as separate at a distance of only 20 to 50 nm apart (a factor of 5 to 10× better). However it requires thousands of images to calculate where the objects are exactly.
Volumetric and multidimensional imaging
Another challenge is presented when producing volumetric images or data sets of biological material and live specimens. Conventional optics only allows for the development of two-dimensional images, but volumetric imaging capabilities are a key requirement in many areas of biological and biomedical research. This requirement goes beyond the conventional “relatively” two-dimensional imaging of cells grown on a cover glass, and moves into the volumetric imaging of tissues and organoids. Volumetric imaging requires a means of detecting objects having been fluorescently labeled from different depths of the tissue, rather than only the surface plane/area.
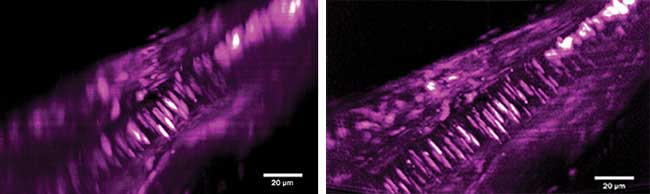
X-Z maximum intensity projections of a volumetric image of a section of fixed tissue from the tail of a juvenile amphioxus (Branchistoma lanceolatum) labeled with propidium iodide to reveal nuclei. The morphological differences between the notochord (well-ordered, elongated nuclei) and the surrounding muscle tissue are only clearly resolved with Airy illumination. Gaussian profile light-sheet image stack with maximum intensity projection, and scale bar for size (left). Airy profile light-sheet image stack with maximum intensity projection, and scale bar for size (right). Courtesy of University of St. Andrews, School of Physics and Astronomy – Kishan Dholakia and Jonathan Nylk.
Volumetric imaging is achieved by changing the distance of the specimen and detection objective in stages, selecting the depth by adjusting the focus, and recording each individual step to create a new Z-stack.
Due to the use of digital imaging detectors, a computer with the appropriate software can be used to tell the system when to acquire a volumetric stack. This creates time-lapse data that can be used to observe the development of a living specimen. If the system comes equipped with multiple excitation light sources, then multicolor channels can give yet another dimension.
Optical sectioning
Confocal microscopes can collect very high-resolution information with great contrast by removing out-of-focus information via a pinhole placed in front of the detector. However, because the illumination and detection objective are one and the same (as it is essentially a conventional fluorescent microscope with sophisticated acquisition tools), objects that were not detected were still illuminated. This contributed to higher levels of phototoxicity and photobleaching.
ALSM, as with other light-sheet modalities, circumvents this issue by illuminating only the plane of interest instead of the whole volume, thus reducing phototoxic effects.
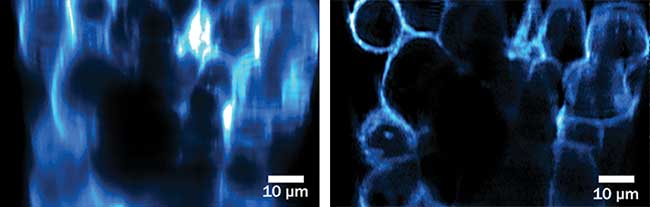
X-Z image slice from a volumetric image of an ACHN cell spheroid, fixed and stained with Alexa Fluor 488 WGA to reveal cellular membranes. Gaussian profile light-sheet image stack with maximum intensity projection, and scale bar for size (left). Airy profile light-sheet image stack with maximum intensity projection, and scale bar for size (right). Courtesy of University of St. Andrews, School of Physics and Astronomy — Kishan Dholakia and Jonathan Nylk.
Another concern with confocal microscopy is the role biological tissue samples have in scattering and absorbing light, thus causing problems in both the illumination path and detection path. The deeper/further the light has to travel, the more likely it is to deviate off course, which causes fuzzy/blurry images. ALSM uses an excitation beam shape that continues on its path (mostly) unimpeded, which is discussed below.
Beam breakdown
There are three principle types of beam that have been used in light-sheet microscopy: Gaussian, Bessel and Airy. Both the Bessel beam and the Airy beam are significantly different from the Gaussian beam light sheet.

A diagram of the beam path of a confocal microscope, the pinhole in the detection path blocks any out-of-focus light from around a diffraction limited spot (down to 200-nm laterally, and 600-nm axially). Courtesy of Danh, CC BY-SA 3.0.
By producing a very thin, uniform sheet of excitation laser light (hundreds of nanometers to a few micrometers thick), fluorophores from the specimen are only excited in the plane of the light sheet. This technique of volumetric imaging is known as Gaussian light-sheet microscopy. In this type of microscopy, the sample can be stepped along the detection axis at varying distances, building up a multidimensional volumetric image of the specimen with digital assistance.
Both the Bessel beam and Airy beam generate intricate, yet distinct, patterns of light near the central order beam. Both penetrate deeper through the specimen than a Gaussian beam.
Each type of waveform operates in a different modality, distributing energy in different positions and intensities along the beam. The amount of energy and the peak irradiance of each form of emission is crucial in understanding the setbacks and trade-offs in light-sheet microscopy and the reasons why ALSM yields benefits that other forms do not.
Gaussian light sheets can resolve the smallest details, producing the crispest volumetric images. However, geometrical limitations prevent the Gaussian light sheet from providing high-resolution light-sheet volumetric images. Its high-resolution images are produced within a limited FOV, owing to the beam’s restrictive Rayleigh range. Increasing the FOV is done by lowering the numerical aperture of the beam, which results in compromising axial resolution and the quality of the final image.
Furthermore, a greater exposure time — or peak irradiance — is required to produce an image at the limits of depth penetration, resulting in greater saturation for some areas of the image and the loss of information altogether in some regions of the final image. This means that, though the Gaussian light-sheet produced the first considerable time savings in producing volumetric images of the same sample size, it is not a viable alternative to confocal scanning. Such an alternative would need to penetrate to a greater depth without providing harmful effects on the specimen or saturation of the image.
Peak irradiance for Bessel and Airy beams is only a fraction of that of the Gaussian light sheet, giving them greater penetration at lower laser power levels, sparing both the phototoxic effects of irradiation on the sample, and the greater levels of saturation in the resulting image.
Similarly, the FOV of the optical system of both Bessel and Airy light sheets is determined geometrically by the numerical aperture along with other factors, and corresponds to the amount of recorded data that can be deconvolved and turned into a useful, high-resolution image.
The Bessel beam is propagation-invariant, solving the Gaussian beam’s issues of divergence, widening the possible field of view. One researcher noted, “Propagation invariant light fields such as Bessel beams can create a thinner light sheet but the transversal outer ring structure of the Bessel beam produces background fluorescence and precludes high axial resolution unless two-photon excitation is used. Single-photon Bessel beam approaches can reclaim contrast using structured illumination or confocal scanning, but the transversal structure of the Bessel beam still unnecessarily irradiates the sample.”1
Advantages of Airy light-sheet microscopy
Airy light-sheet lobes can carry the optimal pattern of energy through the sample area to produce a clean, crisp volumetric image without photobleaching or phototoxic effects after post processing/deconvolution.
Airy beams propagate as bent lobes, resulting in considerable distortion in the recorded image. Since the airy light sheet propagates in lobes, rather than beams, the blurring effects that restrict the Gaussian beam’s FOV are avoided. Powerful algorithms can then deconvolve this image, with uniformly effective results across the field of view.
The Airy light sheet also retains higher spatial frequencies on propagation, unlike the Bessel beam, so the Airy light sheets extend through the sample field in a uniformly thin emission, ensuring optical uniformity in the image quality (isotropic resolution), even when close to the diffraction limit. The field of view has been considerably increased, without losing the high resolution provided by the Gaussian beam.
The lobes issued by the Airy light-sheet microscope reach far lower peak irradiance and can therefore penetrate far deeper sample areas with minimal photobleaching, providing the highest quality volumetric image of the sample field.V
As a result, the Airy light-sheet microscope can collect a large number of volumetric images at a rapid rate of acquisition and at high resolution, with minimal photo-toxic effect on the specimen. This opens up the possibility of the live imaging of delicate samples, for much longer time periods, that would be otherwise destroyed or at least badly damaged by laser scanning confocal microscopy or conventional fluorescence microscopy.
Reference
1. T. Vettenburg et al. (May 2014). Light-sheet microscopy using an Airy beam. Nat Methods, Vol. 11, p. 541.
Meet the authors
Kishan Dholakia, Ph.D., is a professor at the School of Physics and Astronomy, University of St. Andrews, Scotland, and research fellow of the Royal Society of Edinburgh; email: [email protected].
Jonathan Nylk is a research fellow at the University of St. Andrews, and recently completed his Ph.D. His research focuses on the use of optical beam shaping in microscopy; email: [email protected].
Graeme Malcolm, Ph.D., OBE, is CEO and co-founder of M Squared Lasers Ltd. Earlier this year he was awarded an OBE (Order of the British Empire) for his services to science and innovation; email: [email protected].
Robert Forster is general manager at M Squared Lasers. He was formerly director of Acutius Ltd. and general manager for Nikon UK Ltd.; email: [email protected].
Pete Pitrone is a microscopy specialist who formerly worked at the Max Planck Institute forMolecular Cell Biology and Genetics in Dresden, Germany; email: [email protected].