Today’s aging population faces an increase in chronic afflictions such as rheumatoid arthritis, cancer and cardiovascular disease. Photoacoustics is a novel imaging technique that could help monitor disease at an early stage, providing structural and functional information of blood vessels and tissues. Efforts are underway to drastically shrink the size of existing photoacoustic imaging systems by developing highly efficient ultra-short pulse emission near infrared laser diodes.
CELINE CANAL AND ANDREAS KOHL, QUANTEL
Photoacoustic imaging (PAI) has attracted much attention in recent years as a complementary technique to classical ultrasound and optical coherence tomography. This novel technique combines pulsed laser light for excitation of tissues with an ultrasound transducer as a receptor. PAI penetrates deeper than pure optical imaging technologies, such as optical coherence tomography, and offers far greater specificity than conventional ultrasound imaging. PAI has the ability to detect hemoglobin, lipids, water and other light-absorbing chromophores by changing the laser source wavelength. In addition to high-resolution visualization of anatomical structures, such as the microvasculature, multiwavelength PAI provides functional information, such as blood oxygenation, blood flow and temperature. This aspect makes PAI attractive in a wide variety of applications in clinical medicine, preclinical research and basic biology.

Figure 1. A four-wavelength laser source providing 1.3 mJ per wavelength in pulses of 90 ns. Photo courtesy of Quantel.
In combination with ultrasound imaging, PAI can deliver real-time, high-resolution images showing small structures that conventional acoustic or optical scanners would miss. That degree of resolution makes this technology particularly suitable for primary health care, avoiding unnecessary, costly, hospital-based scanning methods, such as MRI and CT. Currently available PAI systems are usually based on nanosecond lasers such as Nd:YAG lasers or optical parametric oscillators. As such, one of the hurdles for the introduction of PAI into the clinic, or even into clinical pilot studies and larger trials, is the bulkiness and price of the laser source.
A need to adapt
Not only is the population aging, but people are living longer. Inevitably, this will lead to more hospitalizations and clinical interventions. As a result, health care delivery needs to adapt by providing more cost-effective medical care in early disease detection, improved diagnostics and therapy monitoring through point-of-care functional imaging. Portable imaging devices have grown in importance due to the numerous benefits they offer1. They simplify hospital workflows and can easily be shared between care centers. This helps reduce costs and offers medical accessibility to patients who cannot be seen in an office, such as those under home care or in underdeveloped areas.
The challenge is to reduce the size of the laser source in a photoacoustic scanner so that the scanner is small enough to be taken directly to the patient. Ideally, this laser source should fit into a handheld device about the same size as a classical ultrasound head today.
Technical challenges
The most compact laser source available today is a laser diode. For an effective photoacoustic system with a sufficient spatial resolution, short laser pulses with a width of less than 100 ns are required. To achieve a significant penetration depth, the pulse energy should be at least 1 mJ at a pulse frequency of typically 1 kHz. A pulse energy of 1 mJ in a 100 ns pulse means an optical peak power from the diodes of 10 kW.
The Quantel laser diode source is made of high power diode bars — 10-mm wide strips of semiconductor typically containing 40 individual emitters in parallel. The conversion of electrical current to optical power by each bar is typically about 1 W of optical power per 1 A of drive current. To allow both the laser source and the ultrasound transducer to fit into a handheld device, only 10 or fewer diode bars can be used. Therefore, each diode bar must provide at least 1 kW of optical power. This means that a drive current of 1 kA is required to achieve the 1 kW peak power per diode bar. Handling nanosecond pulses with kA currents in the very confined volume of a handheld device — without generating significant waste heat — was the primary challenge in this development.
Single wavelength devices
To provide compactness and efficiency, the laser diodes had to be integrated directly on the electronic driver board. A complete redesign of the driver electronics was necessary to handle the high peak currents in short pulses. Heat generation was minimized by using highly efficient diode bars and eliminating electrical losses.
Electrical connections between a diode and the driver board were finely optimized to keep the inductance of the connectors at the lowest possible level. Since 2011, when the first laser diode module prototype was built as a proof of concept, efforts focused on increasing the output energy while reducing the laser pulse width. The goal was to provide high peak power in the range of 10 kW.
At this stage of development, only one wavelength per laser source was available, at about 808 nm, a wavelength easily absorbed by blood cells. Demonstrations of low-cost, portable and sensitive photoacoustic systems integrating Quantel laser diodes were performed with in-vitro and in-vivo experiments.2,3 These laser diode sources typically provided 1 mJ in a 135 ns pulse (full width at half maximum). With an overall efficiency of 25 percent, including the driver efficiency plus the diode bar electro-optical conversion, the heat generated at a repetition rate of 1 kHz was about 3 W. This low heat level is fully compatible with a handheld device.
Multi-wavelength laser sources
Multi-wavelength laser diode sources are a real asset in tasks such as the differentiation of tissues or the quantification of blood oxygenation. Figure 1 shows a laser diode with a laser source that can integrate up to four diodes in a tight volume, with each diode having a different wavelength in the range 808 to 980 nm. Design of a device with multiple diodes — and that can fit into a single assembly while reducing the connector’s inductance to provide shorter pulses — posed a significant challenge. Compactness of the packaged diodes needed to be achieved while providing different wavelengths that may be switched on independently or all together. Each diode is connected to a pulse generator, with all four generators located on the same driver board.
The diodes each emit 1.3 mJ output energy in pulses of about 90 ns, reaching more than 14 kW peak power per wavelength. Any combination of one, two, three or four wavelengths can be used, provided that the average power in the 1 cm2 output does not exceed 2 W of optical power. That is a limit for patient safety reasons. Pulse bursts at a maximal frequency of 10 kHz are still possible if the burst duration is short enough to comply with safety regulations. The overall electrical-to-optical efficiency is higher than 25 percent for all wavelengths.
A demonstration of four simultaneously generated wavelengths was performed with a prototype that provided wavelengths of 808, 915, 940 and 980 nm (Figure 2). Up to 5.2 mJ were recorded with four wavelengths emitting at the same time with all pulses superimposed. Further characterization of this laser source has shown a stable energy during more than 15 × 109 shots (15 Giga-shots) at a frequency of 2 kHz. These results show that despite the high power and energy, the reliability is sufficient to ensure many years of operation in a medical device.

Figure 2. Normalized spectra of a four-wavelength laser source. Photo courtesy of Quantel.
The output beam from a diode is elliptical due to divergence in the horizontal and vertical axes, with a respective value of 11° and 65° (full width angle at 1/e2). Given that 65° is much too large to provide high power density on the target, each diode bar was collimated in the vertical axis with cylindrical micro-lenses to reduce divergence to about 2°. A custom optical system can be added to further shape the beam for Gaussian or quasi-top-hat profiles in both axes. Typical output beam size from each of the photoacoustic scanner’s wavelengths can be 20 × 5 mm2 on the skin.
Toward shorter pulses
One way to reduce the pulse width, while maintaining the pulse energy at a mJ level, involves thoroughly reducing self-inductance on the driver board and in the connections between the driver board and the diode. Doing this will lead to the laser source shown in Figure 3. The laser source has an extremely small footprint of 40 × 50 mm2, providing up to 1.7 mJ pulses of about 40 ns width.
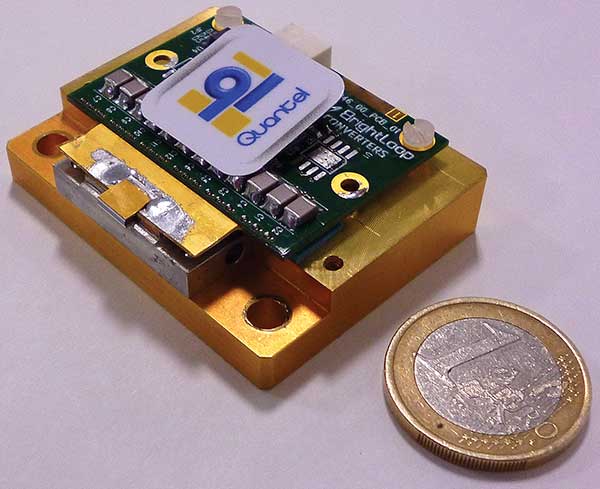
Figure 3. An ultra-short pulse laser source integrating a mini-laser diode providing 1.7 mJ in pulses as short as 40 ns. Photo courtesy of Quantel.
This laser source was built with 5-mm diode bars instead of 10-mm length. This presents two advantages: 1. Reduced price, due to the decreased number of emitters; and 2. Reduced output beam ellipticity to better enable fiber coupling. Miniaturization of the diode led to an emitting surface as small as 6 mm2 (5 × 1.2 mm2) compared to the standard value of 22 mm2 (10 × 2.2 mm2). This assembly provides 1.7 mJ output energy in pulses of 40 ns at a wavelength of 808, 915, 940 or 980 nm (Figure 4). This short pulse width eclipses a state-of-the-art mJ pulsed laser diode. Energy and pulse width can be easily configured by adjusting the number of diode bars and capacitors on the driver board in which electrical energy is stored before current pulse generation. Further work on this mini-laser diode will focus on coupling light into a multimode optical fiber.

Figure 4. A 40-ns optical pulse recorded from an ultra-short laser source. Image courtesy of Quantel.
Conclusion
One key to development of the low-cost, portable devices so highly needed for point-of-care testing is the introduction of laser diodes in such systems. Major progress has been made to bring mJ laser diodes into the nanosecond laser class where previously only bulky and expensive lasers were available.
For example, Quantel’s laser sources, with a footprint of 20 cm2, provide up to 1.7 mJ with pulses as short as 40 ns at a single 808, 915, 940 or 980 nm wavelength. Repetition rate can be as high as 10 kHz, providing an average power of a few watts. The high efficiency of these laser sources enables operation at 1 kHz without cooling. A multi-wavelength laser diode version can have up to four wavelengths in a highly compact package delivering pulses shorter than 90 ns. This is a real asset for contrast enhancement in medical imaging. The multi-wavelength laser sources also showed an overall efficiency higher than 25 percent. The generated waste heat is low enough to be fully compatible with a handheld device. Such laser sources can be used for ultrasound imaging for medical applications.
Meet the authors
Celine Canal is a project manager and leader in the research and development department at Quantel in Les Ulis, France; e-mail: [email protected]. Andreas Kohl is the head of operations for laser diodes for Quantel; e-mail: [email protected].
Acknowledgments
Part of the research leading to these results has received funding from the European Commission’s Seventh Framework Programme (FP7/2007-2013) under grant agreement n° 318067.
References
1. M. Freebody (October 2015). Portable imaging gets high-tech. BioPhotonics, pp. 28-31.
2. K. Daoudi et al. (2014). Handheld probe integrating laser diode and ultrasound transducer array for ultrasound/photoacoustic dual modality imaging. Opt Express, Vol. 22 (21), pp. 26365-26374.
3. P.K. Upputuri et al. (2015) Performance characterization of low-cost, high speed, portable pulsed laser diode photoacoustic tomography (PLD-PAT) system. Biomed Opt Express Vol. 6 (10), pp. 4118-4129.