Emerging adaptive optics systems aim to multiply degrees of freedom, pixel counts, and photosensitivity while bringing system costs down.
HANK HOGAN, CONTRIBUTING EDITOR
Adaptive optics (AO) technology enables Earth-based telescopes to take the twinkle out of the stars. The technology also helps in detecting and reversing eye disease, it improves the throughput of laser-driven manufacturing, and it has enabled the world’s most powerful lasers to achieve peak power. AO achieves these improvements by removing distortions endemic to optical wavefronts, thereby ensuring the clearest possible imaging, the highest laser power, and maximum manufacturing efficiency.
But adaptability is a moving target, and AO technology can always improve. End users are always seeking solutions that are able to provide quicker wavefront corrections and removal of finer aberrations. They also want lower-cost options. Technology suppliers are adapting their AO solutions to address these issues.
The operation of an AO system begins with wavefront sensors that determine how an incoming wavefront of light deviates from an ideal flat plane. The deformable mirrors then alter the shape of their reflecting surface so that the reflected light is as close to the ideal as possible. Deformable mirrors can alter their shape with varying degrees of freedom, determined by the number of actuators that they incorporate.
AO systems can alternatively correct the wavefront using spatial light modulators (SLMs). These components commonly employ liquid-crystal-on-silicon (LCOS) technology in which light passes through a liquid crystal layer that sits atop an array of pixels driven by electronic components. The electronics can change the optical properties of the liquid crystal layer to modulate the time delay or phase of the light — on a pixel-by-pixel basis — as it passes through the liquid crystal and is reflected out again. The result is an outgoing wavefront with little or no aberration compared to the incoming beam.
Clearing the atmosphere
Astronomy applications, in particular, have pushed the boundaries of adaptive optics over the years, according to Simon Thibault, a physics professor at the Laval University in Québec City. His experience with AO technology spans more than 20 years and, today, Thibault develops instrumentation for astronomy and microscopy researchers. As with other AO developers, he and his team rely on an assembly of wavefront sensors, as well as deformable mirrors or SLMs.
In Earth-based telescopes, wavefront aberrations arise from atmospheric turbulence. The emergence of ever-larger telescopes, such as the 30-m giants currently under construction, is pushing AO technology to evolve further to support these state-of-the-art instruments.
“You need more and more degrees of freedom on the deformable mirrors or spatial light modulators — the elements that will correct for the wavefront,” Thibault said. “The other thing you need is the speed.”
In other words, astronomers need deformable mirrors with more actuators or SLMs with more pixels. Either option would increase the degrees of freedom in an AO system. Faster correction speeds are particularly important to Earth-based telescopes, which require thousands of wavefront corrections to occur every second, Thibault said.
Applications in microscopy or ophthalmology, such as imaging of the retina, can get by with correction rates that are an order of magnitude slower, but these rates still qualify as rapid imaging, and they are particularly important when capturing what is happening in a living cell.
Astronomy is also testing the limits of adaptive optics with the growing study of planets that orbit other stars. Telescopes can only spot these exoplanets by blocking out the light from the planets’ parent star by using an instrument called a coronagraph, which requires AO technology to make the star’s image appear still. AO technology also helps to sharpen the images of the exoplanets themselves.
Among the companies developing AO technology to make such applications
possible is Boston Micromachines Corp. According to Michael Feinberg, the company’s vice president of sales and marketing, its deformable mirrors rely on MEMS actuators, which are fabricated by applying a continuous reflective thin film on top of miniscule mechanical components. The actuator mechanisms push and pull on the thin film to form tiny hills and valleys that correct for the distortions in the wavefront of incident light.
Boston Micromachines has developed a deformable mirror with over 4000 actuators. For exoplanet research, it is developing another with 8000 actuators, Feinberg said. Microscopy applications, in comparison, typically require only 100 to 200 actuators.
The first direct image of two giant exoplanets orbiting a sun-like star some 300 light-years away, taken by the European Southern Observatory’s Very Large Telescope. The planets are fairly young and, consequently, shine brighter in the infrared spectrum. With advancements in adaptive optics (AO), scientists may be able to image smaller and older planets orbiting closer to the parent star. Courtesy of ESO.
Because the film is thin and stiff and the actuators are small, the company’s deformable mirrors can operate very quickly, with a mechanical response time as short as 20 ms.
Boston Micromachines is working on making this time even shorter by further refining the MEMS and mirror components. It comes down to structural design, Feinberg said. “A big factor is air damping.”
Other innovations in the works focus on advancements in thin-film coatings. By nature, many astronomy, microscopy, and laser communications applications are starved of photons. So getting the maximum amount of light through an AO system is critical. One way to accomplish
this is to increase the reflectivity of the thin-film mirror component, which requires the right combination of coatings.
While these coatings may change the composition of the film, care must be taken to maintain the film’s ability to flex many times without failing.
Adaptive optics is also instrumental in applications characterized by an abundance of photons, such as in laser-based materials processing or in high-intensity laser research.
Light-sheet imaging of live fruit fly brain neurons involved in the circadian clock network, captured at a depth of 45 µm. Without AO (left) and with AO (right), which enabled a marked improvement in image clarity. Scale bar = 20 µm. Courtesy of Imagine Optic.
An example of the latter can be found in Europe, where deformable mirrors from Imagine Optic Inc. play a key role in the 10-PW Extreme Light Infrastructure in Magurele, Romania. The high-intensity lasers developed at this beamline facility achieve their extreme output powers by first expanding pulses of light in time and space. The deformable mirrors eliminate distortions in the wavefront before the pulses are then compressed again, leading to a peak power in the petawatt range.
Adapting for resolution
Imagine Optic also makes Shack-Hartmann wavefront sensors that operate in the visible and shortwave IR. The sensors determine the wavefront shape by sending the light through an array of microlenses that focus it onto a sensor. Sending the location of the resulting spots through an algorithm helps to determine the wavefront of incoming light and the information needed to adjust an AO system’s deformable mirror or SLM.
A typical Shack-Hartmann setup leverages only the center of each spot. Imagine Optic has developed a technique, called LIFT, that also uses the shape of the spot to adjust the system’s active elements.
Deformable mirrors, such as this 8000-count actuator model from Boston Micromachines, adjust their shape to counter wavefront distortions in light. Courtesy of Boston Micromachines.
“When the shape of the spot is not symmetrical, then that means the wave in front of the microlens is not a plane,” said Xavier Levecq, chief scientific officer at the company.
With this additional information, the AO system’s software can do a better job of profiling the wavefront to achieve a sixteenfold improvement in spatial resolution. Experiments by Imagine Optic indicate that this increased resolution leads to visibly improved optical metrology results.
Compared to standard approaches, the technique requires more computation, which slows down the response time of the adaptive optics. Levecq said the company is porting the algorithm from a general processor to GPUs, which should help mitigate the issue significantly. The aim is to achieve a fast enough response time for the technique to be useful in microscopy and ophthalmology applications.
A closer look at SLMs
Today’s state-of-the-art SLMs may incorporate an array of 1900 × 1200 pixels, according to Kipp Bauchert, vice president of SLM technology development at Meadowlark Optics Inc. One constraint on the pixel count is the distance that must be maintained between adjacent pixels, which is determined in part by characteristics of the liquid crystal layer.
“When the pixel pitch starts approaching approximately 2× the thickness of the liquid crystal layer, you start having electrical field fringing or crosstalk,” Bauchert said.
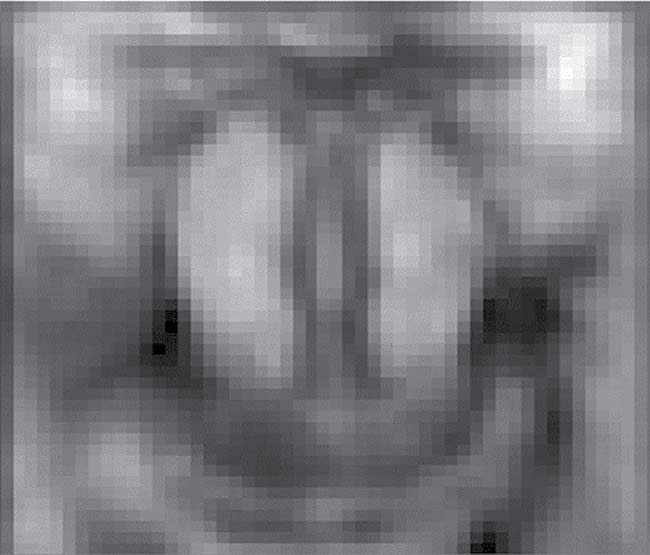
An image of a mandrill, corrected by conventional approaches (top) versus the image corrected by a technique called LIFT (bottom). Shack-Hartmann sensors used in AO systems typically only leverage the center of each variation in a wavefront. The LIFT technique also uses the shape of the spot to adjust the AO system’s active elements. The result is a sixteenfold improvement in spatial resolution. Courtesy of Imagine Optic.
The response time of the liquid crystal material depends on the wavelength of the incoming light and its polarization. As a result, maximum AO correction rates are a few hundred hertz, which is fast enough for all but the most demanding applications.
Additionally, the composition of liquid crystal permits light only of a particular polarization to pass through to the pixel layer, which means that it loses half of the photons in randomly polarized light. This loss is an issue only if an application is light-starved to begin with.
While SLM-based AO systems remain useful for many imaging applications, they are well suited for optimizing the beam in laser processing operations. Adaptive optics can enable a single ultrashort laser pulse to be split into as many as 16 pulses, for example, said Columbine Robinson, applications engineer with Hamamatsu Corp.
A liquid-crystal-on-silicon spatial light modulator. The liquid crystal material used in many spatial light modulators only permits light of a particular polarization to pass through to the pixel layer, which means that half of the photons in randomly polarized light are lost. While this quality may present challenges in light-starved imaging applications, the technology is well suited for optimizing the beam in laser processing operations. Courtesy of Hamamatsu.
“The parallelization spots are used for laser machining of silicon,” Robinson said. “The limiting factors in the parallelization are the optics used and the required power density at the focal point.”
Thus, the throughput of one beam for certain manufacturing tasks can be substantially increased up to a maximum 16:1 ratio. With cooling, Hamamatsu’s 1272- × 1024-pixel SLMs can handle laser powers up to 100 W, according to Robinson.
Brad Coyle, LCOS-SLM product manager at Hamamatsu, said that SLMs equipped with fast processors, such as field-programmable gate arrays, provide the speed, resolution, and feedback required to enable real-time aberration correction.
Coyle said he expects to see AO systems in the future that are able to handle higher power.
Innovations are also needed, Boston Micromachines’ Feinberg said, to drive down system costs and thereby push the technology into higher-volume applications and wider use. Such advancements could be enabled by leveraging semiconductor manufacturing practices to fabricate AO systems. “As we increase the yield of our devices, and as the volume of those devices goes up because they’re in more instruments,” he said, “we will see the costs drop.”