Photonics could potentially be a key technology in the emerging market of laser space communications, with unique performance characteristics. It may enable new bandwidth-hungry applications and significantly boost the space communications industry downstream.
EFSTRATIOS KEHAYAS, GOOCH & HOUSEGO
Photonics is rapidly transforming into an enabling space technology with potential to cross-fertilize multiple markets in the space domain. Among them: navigation, remote sensing and telecommunications, as well as ground-breaking scientific and planetary exploration missions. Because of advantages related to bandwidth, mass, power consumption, beam size and immunity to electromagnetic interference, photonic subsystems are now being considered in navigation satellite systems, Earth observation satellites, low Earth orbit (LEO) constellations and within telecom satellite payloads1.
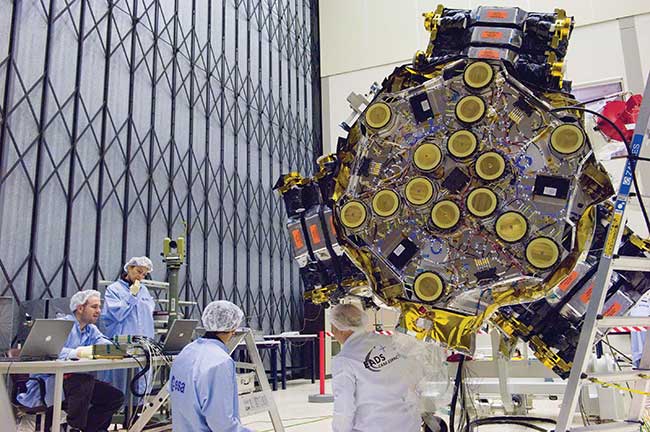
The European Space Agency’s Soil Moisture and Ocean Salinity (SMOS) satellite MIRAS instrument shown during testing in 2007. The transmission of the master clock signal, the local oscillator and the received digitized data is performed with an optical fiber digital network called MOHA. Courtesy of A. Le Floc’h/European Space Agency.
Token examples of functionalities being demonstrated in the laboratory and in space include stable laser sources, high-speed transmitters, highly sensitive receivers, on-board optical processing systems and optical clock distribution. A key milestone in the robustness of photonics was the 2009 deployment of 1.55-μm fused fiber couplers on a mission-critical payload2 onboard the European Space Agency’s (ESA) Soil Moisture and Ocean Salinity (SMOS) satellite. The nominal lifetime of the satellite was three years (including a six-month commissioning phase). But now, more than seven years later, SMOS remains fully operational and continues to gather information3.
Communication terminals
Laser communications between satellites or from satellite-to-ground can enable high-speed connectivity with reduced spacecraft resources in terms of mass, real estate and power4. Space-based laser systems rely on 1064- or 1550-nm wavelength windows for establishing high-capacity networks from satellites on the low Earth5 or geostationary orbits6,7. This allows for piggybacking proven terrestrial photonic technologies and, where required, exploiting wavelength division multiplexed technology for increasing the aggregate channel capacities.
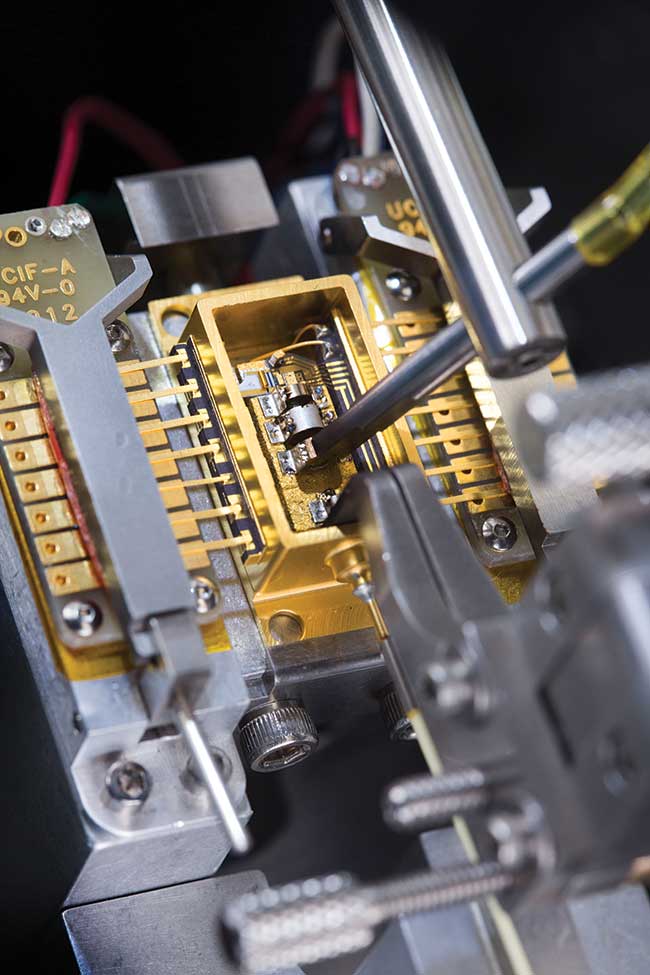
High-power distributed feedback laser used during assembly as the light source within laser communication terminals. Courtesy of Gooch & Housego.
A laser communication terminal (LCT) is the space equivalent of a transponder in line-side equipment of terrestrial networks. The LCT is usually responsible for “preparing” the data and “establishing” the communication link. Although standardization on laser communications has been initiated within the Consultative Committee for Space Data Systems (CCSDS), to date there is no standard transparent system definition and no multisource agreements for space photonic hardware.
Nevertheless, the laser terminal typically performs Layer-1 and Layer-2 functionalities as per the traditional OSI reference model. In terms of functional blocks, such a terminal is composed of a central processor, a fiber optic transmitter and receiver subsystem, and a free space assembly for performing pointing acquisition and tracking. Vital photonic components for realizing the fiber optic transmitter and receiver subsystem include lasers, electro-optic modulators, amplifiers and photodetectors for the generation, coding, amplification and reception of light, respectively.
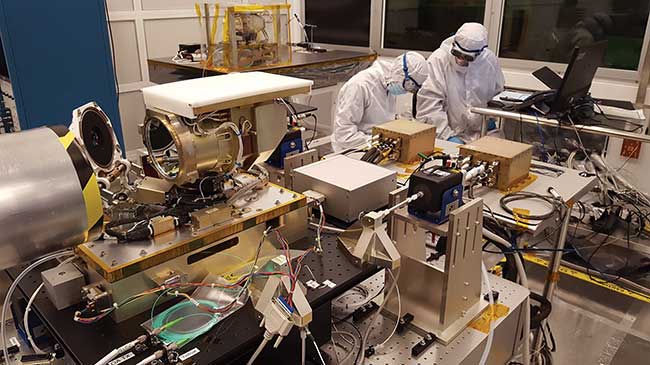
Work is performed on an optical module of the Laser Communication Relay Demonstration (LRCD) optical module, which consists of a gimballed, inertially stabilized telescope with a 10.7-cm diameter aperture. Courtesy of NASA Goddard Space Flight Center, with thanks to Dr. Donald Cornwell for securing approval.
The photonic components deployed in space require additional performance evaluation when compared to conventional terrestrial telecommunication counterparts. The key parameter to be defined is the end-of-life performance when subjected to worst-case degradation factors. In certain cases, Telcordia-qualified components can be designed into a system with additional customization, further space assessment and up-screening. In other cases, a completely new design of the component is required because of the inherent limitation of the materials used or the manufacturing methods of the equivalent conventional terrestrial components. Most importantly, however, the components, modules and corresponding systems need to be able to operate under the presence of radiation. The Earth’s orbit is an inhospitable environment for electronics and photonics.
Satellites orbiting our planet usually do so within or close to the inner and outer Van Allen belts when they are deployed in the low Earth and geostationary orbits, respectively. Within these areas, because of the Earth’s magnetic field, transit galactic and solar cosmic radiation is trapped. The radiation consists of electrons, protons and heavier ions. Depending on the orbit in which the satellite is deployed, different types of radiation are present; hence different degradation mechanisms are activated. To make conditions more challenging, the effect of this orbit-dependent radiation differs according to the component characteristics, e.g., its material system and feature size.

An engineer works with a high-power laser diode subassembly for satellite communications. Courtesy of M. Tuci/Gooch & Housego.
As such, a key milestone within the development phase of any space photonic hardware is the quantification of the performance degradation against the different types of radiation; this calculates the end-of-life performance to be used in the system worst-case analysis.
Space-grade fiber optics
Optoelectronic components are key building blocks of satellite laser communication systems and intra-satellite links within telecom satellite payloads. Semiconductor lasers are typically used for the generation of light at the preferred wavelength used for transmission, and the optical pump light used in doped fibers or glasses for signal generation and amplification8. In terms of build design, the lasers need to be hermetically sealed and qualified against mechanical, thermal and radiation environments to guarantee survival during the launch and operation phases. In addition to well-defined and tightly controlled manufacturing processes, lasers for space require fully traceable material and component lists in order to ensure compliance with mission-dependent product assurance requirements.
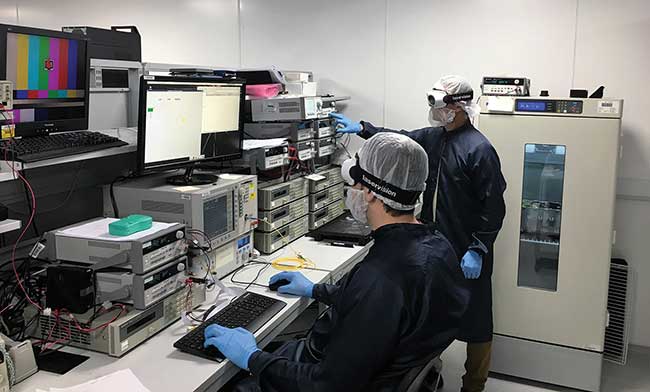
Scientists work on functional performance verification of high-power amplifiers under temperature for LEO/GEO inter-satellite link. Courtesy of Gooch & Housego.
Protecting efficiency
Nonionizing radiation can be detrimental to optoelectronic components, as displacement damage is introduced within the lattice structure. If defects are introduced, they can lead to nonradiative recombination, reducing the efficiency of the device. In laser diodes, the degradation displacement damage is usually manifested as a threshold current shift. In photodetectors, the degradation is typically expressed as a drop of the responsivity — the amount of electrical current generated by a given incident number of photons.
Within the frames of the European Commission’s Horizon 2020 project HIPPO9, distributed feedback (DFB) lasers, high-speed detectors and pump lasers have been developed. Measurements performed on DFB lasers revealed a <5 percent threshold shift at the limiting case of cumulative fluence of 1012 p/cm2 using 60-MeV energies. The high-speed receiver responsivity decreased by <3 percent, while the dark current increased from 40 nA to 50 nA. Minimal to no
degradation was observed by both high- and low-brightness pump lasers; for cumulative fluence levels of 1010 p/cm2 and 1011 p/cm2, which are representative levels for geosynchronous orbit (GEO) and LEO scenarios, minimal degradation is present (<1 percent threshold shift and responsivity degradation for the transmission and photo receiver, respectively).
Fused fiber components are necessary building blocks of various communication subsystems and used to combine, split and filter light within fiber optic assemblies. In addition to robust thermomechanical performance requirements against vibration and shock, passive components are required to operate in thermal vacuum and exhibit radiation hardness. In the case of silica-based fiber optic components, radiation degradation appears in the form of color centers, effectively introducing propagation loss in the signal. Gamma radiation tests following ESA standards (ESCC 22900) that were performed on G&H polarization-maintaining (PM) and non-PM fused couplers and pump combiners showed 1 to 4 percent degradation in insertion loss at the limiting case of 100 krad total ionizing dose (TID).
System integration of high-reliability space-compatible components into functional systems of the LCT requires verification of unit-level assembly processes. Experimentally verifying the radiation performance of optical amplifiers requires a well-thought-out testing strategy and hardware configuration to generate realistic results representative of the target specification.
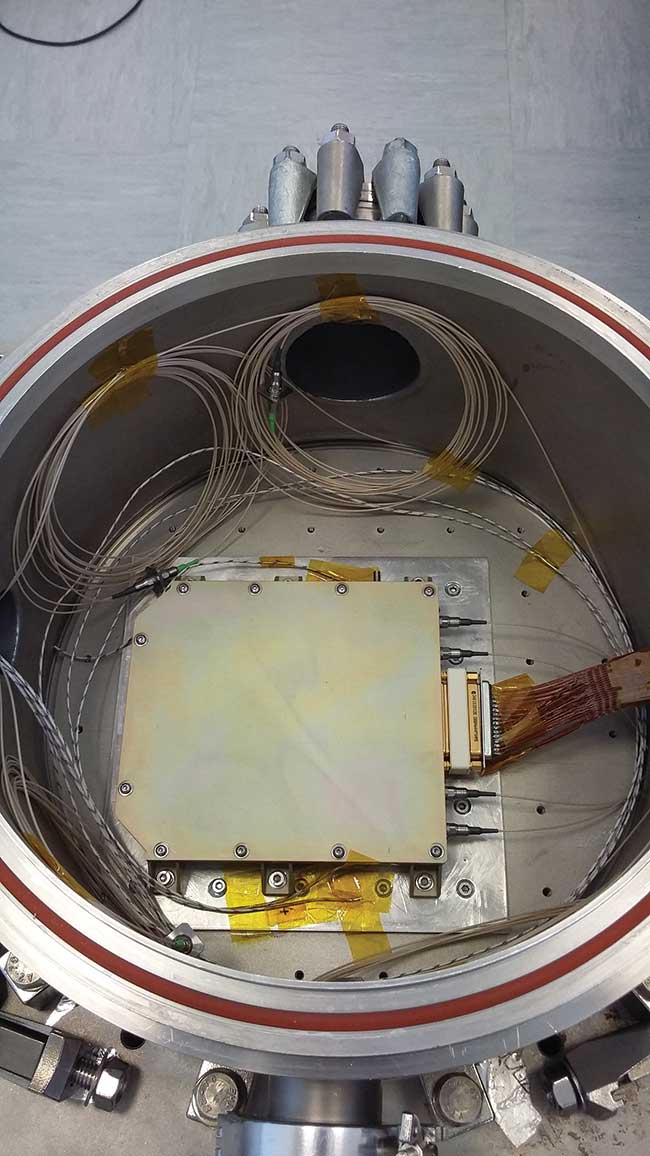
This optical amplifier unit for low Earth orbit-to-ground direct downlinks is ready for temperature cycling in vacuum. Courtesy of Gooch & Housego.
The combination of complex gain dynamics in optical amplification within active doped fiber and radiation-induced absorption can lead to erroneous and often misleading results if the active fiber is not tested in a correct amplifier configuration, thus masking the real degradation. Moreover, the test parameters can also introduce significant variations, skewing the true performance.
Space-qualified systems
Integration of fiber optics and lasers into subsystems can be validated by successfully passing space-level unit tests as per ESA or NASA standards, such as the ECSS-E-10-03, which provides standard environmental and performance test requirements for a space system and its constituents. Typically, in addition to full functional performance verification and electromagnetic compatibility (EMC) testing, a subsystem must pass thermomechanical tests that include shock, vibration and thermal cycling in vacuum. Tests with that particular sequence are specifically defined to mimic the process of launch and in-orbit operation, hence invoking activation of any failure or degradation sources during qualification.
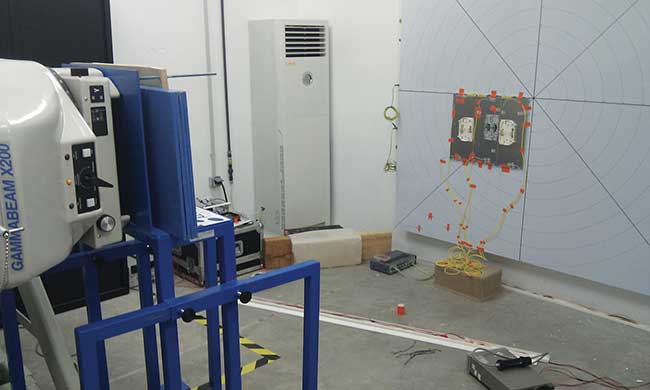
Optical amplifier subassemblies being irradiated using a Co60 source at ALTER and CNA, a testing company and testing facility (respectively) in Seville, Spain, to measure optical gain drop, as well as noise figure evolution up to 100-krad total ionizing dose. Courtesy of Gooch & Housego.
G&H has followed the ECSS unit-level plan to space qualify mid-power booster amplifiers for direct downlinks. Specifically, a polarization maintaining optical fiber amplifier gain block10 was manufactured, which included G&H HI-REL fused and opto-electronic components. The unit survived 2500-g shock and 19-g rms vibration. There were no failures during operation in a vacuum, verifying that unit-level assembly and integration processes are suitable for space applications. Following the verification of the gain block, a complete multichannel amplifier system, including space-qualified electronics, passed a 1000-g mechanical shock, 19.3-g rms vibration and thermal cycling in vacuum, and is ready for an in-orbit demonstration.
If optics and photonics is to penetrate the space sector as an enabling technology, it will need to support the transition of the space industry to Space 2.0, while reinforcing the deployment of cost-effective, inter-satellite networks and mega constellations. This can be accomplished by technology spin-in from other sectors such as telecommunications and industrial systems.
Manufacturing processes of high-reliability telecom applications could be used as a technology baseline, and by adapting process controls and assembly procedures, it can enable high-volume space photonic unit assembly, integration and test.
Meet the author
Efstratios Kehayas, Ph.D., is vice president of R&D at Gooch & Housego, leading space photonics activities; email: [email protected].
References
1. N. Karafolas et al. (March 2009). Introducing photonics in spacecraft engineering: ESA’s strategic approach. Presented at the IEEE Aerospace Conference: Big Sky, Mont.
2. M. Martin-Neira et al. (November 2008). MOHA: the MIRAS optical harness for SMOS. Presented at Photonic Technologies for Beam Forming Payloads, ESTEC.
3. European Space Agency. Surpassing expectations. http://www.esa.int/Our_Activities/Observing_the_Earth/SMOS/Surpassing_expectations.
4. Donald Cornwell (2016). Space-based laser communications break threshold. OSA Optics & Photonics.
5. T. Dreischer et al. (2012). OPTEL-μ: A compact system for optical downlink from LEO satellites. SpaceOps, Vol. 1, pp. 789-798.
6. G. Muehlnikel et al. (October 2012). The Alphasat GEO Laser Communication Terminal Flight Acceptance Tests. Presented at the International Conference on Space Optical Systems and Applications.
7. S. Yamakawa et al. (October 2015). JAXA’s Optical Data Relay Satellite Programme. Presented at the IEEE International Conference on Space Optical Systems and Applications.
8. J. MacDougall et al. (January 2017). Transmission and pump laser modules for space applications. Presented at Free-Space Laser Communication and Atmospheric Propagation XXIX, SPIE Photonics West, paper 10096-16.
9. E. Kehayas et. al, (October 2014). High-power photonics for satellite laser communications and on-board optical processing. International Conference on Space Optics, paper PS2.07.
10. L. Stampoulidis et al. (January 2017). Radiation-resistant optical fiber amplifiers for satellite communications. Free-Space Laser Communication and Atmospheric Propagation XXIX, SPIE Photonics West, paper 10096-15.