Advances in detectors and sources have gotten quantum communication ready for rollout. Photonics plays a key role in this technology, thanks to the ability to impose a quantum state on particles of light and to detect that state at a point many miles away.
Quantum communication may be ready for its big debut, but technical issues – such as how to increase transmission distance or construct a quantum repeater – still must be worked out. There are also questions about the commercial viability of the most near-term application, quantum key distribution (QDK). However, there is a clear need, particularly in highly secure settings.
“Key distribution and key generation are key to the success of any transmission security network,” said Karl Fuchs, vice president of technology for satellite communications vendor iDirect Government Technologies of Herndon, Va. The company sells products and services to the US military and other demanding customers.
The biggest performance hit arises from disseminating the initialization key, the basis for all subsequent encryption, Fuchs said. Consequently, overall system performance and security would improve with faster and more secure key switching. Quantum key distribution achieves the security objective absolutely by taking advantage of the ability to detect an eavesdropper via changes in the measured quantum characteristics of a photon.

Run across Switzerland, a pilot quantum key distribution (QDK) network was the longest running test bed of the technology. CERN = European Organization for Nuclear Research; UNIGE = University of Geneva; Hepia = Engineering School of Geneva. Jet d’Eau is Geneva’s landmark water fountain. Courtesy of ID Quantique.
The operative words here are “a photon,” as in a single photon. Current commercially available quantum key distribution schemes require single-photon sources and detectors. The former can be approximated well enough by attenuating a laser so that, on average, much less than one photon is present in any given clock cycle. That clock rate, in turn, is set by the speed of single-photon detectors, and here, progress has been made in the past few years.
Rapid detectors
For example, Toshiba Research Europe Ltd. of Cambridge, UK, a subsidiary of Japan’s Toshiba Corp., has developed technology that allows conventional avalanche photodiodes to run 100 times faster in single-photon mode than previously possible. The advance involves comparing detector output from the current clock cycle to the one before, said Zhiliang Yuan, the quantum key distribution project leader. This self-referencing removes an unwanted periodic signal that interferes with sensitive detection of single photons, enabling devices to recover faster because the detectors are in a more favorable configuration.
“The detector can be moderately biased for efficient single-photon detection, and the detector recovery time is substantially reduced. We have confirmed that the detector recovery time is less than two nanoseconds,” Yuan said.
These and other developments have made quantum key distribution at acceptable data rates viable, said Grégoire Ribordy, CEO of the Geneva-based ID Quantique SA, a company commercializing the technology.
Unfortunately, the quantum nature of the communication presents other hurdles. For instance, it’s difficult to mix quantum traffic with traditional telecom data. The latter involves a multitude of photons, and crosstalk from these can destroy the single-photon signature.
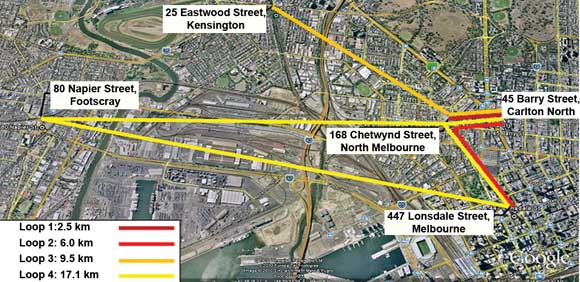
A test conducted in Melbourne, Australia, demonstrated successful coexistence of classical and quantum communication over fiber. Courtesy of QuintessenceLabs.
One solution is to dedicate optical fiber to quantum communication, but keeping fiber dark for all other traffic is expensive. A possible solution is to spread the cost among many users, something that can be done if quantum key distribution is sold as a service by a telecom provider, Ribordy said.
“They would offer an optical network which would be shared by several QKD systems. You can do wavelength division multiplexing,” he said.
He noted that quantum key distribution as a service is now a reality, with the first customer using it announced in December. Another firm involved in applications of the new technology is MagiQ Technologies Inc. of Somerville, Mass. Because quantum key distribution has yet to generate sufficient sales, companies are turning to other revenue streams (see sidebar below, “Making More Money in the Meantime”).

This flow diagram shows the data path of the pilot QKD network in Switzerland. Courtesy of ID Quantique.
Second-generation systems that circumvent these problems also are in development. One is being pursued by QuintessenceLabs Pty. Ltd. of Canberra, Australia. Rather than encoding information on individual photons, the company’s systems perform encoding via the amplitude and phase quadrature of a weakly modulated coherent laser. This approach offers high data rates because the receivers used are basically off-the-shelf coherent homodyne detectors that have a 10-GHz bandwidth, said founding director and CEO Vikram Sharma.
Peaceful coexistence
Another advantage can be seen in a test done with Telstra – Australia’s largest telecommunications carrier – in Melbourne. In the test, one quantum channel operated at one frequency. Running beside it, but at another frequency, was a second channel that carried synchronization and timing information using classical telecommunications.
“It offers the possibility of having multiple quantum channels coexisting with existing traffic,” Sharma said of this proof of successful mixing of quantum and standard communications.
In November 2011, QuintessenceLabs partnered with prime defense contractor Lockheed Martin to showcase its technology. QuintessenceLabs also plans to bring out a commercial product in the third quarter of 2012.
These and other efforts will be aided by the implementation of standards governing quantum communication, another necessity for commercial deployment. The Vienna-based Austrian Institute of Technology GmbH is spearheading the development of standards and guidelines that will outline the use and certification of quantum cryptographic equipment and networks.
Thomas Länger is chairman of a group setting industry specifications for the European Telecommunications Standards Institute. A scientist at the Austrian Institute of Technology, he noted that standard setting is quite demanding. “On the low level, the physics level, this is not so very clear how to tackle this. We need new models for that, new notions for security.”
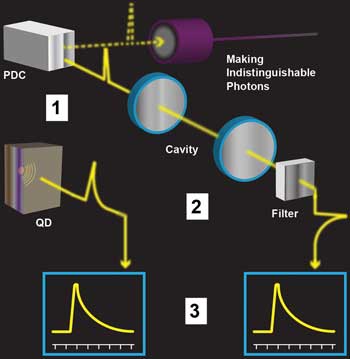
Quantum communication and information processing requires virtually indistinguishable photons. NIST researchers achieved this, producing (1) a single photon using a quantum dot (QD). Simultaneously, they created a pair of photons by a parametric down-conversion (PDC) crystal. (2) One of the PDC photons – which has characteristics different from the QD photon – is routed into a cavity and filter (3), rendering the PDC photon and the QD photon nearly identical. Courtesy of Suplee, NIST.
Länger expects these efforts to bear fruit within a few years. Having standards in place will help convince large firms to take the quantum plunge, either as suppliers or as customers.
Further in the future looms complete quantum communication and information processing, but several devices must first be invented and perfected. For instance, one requirement will be quantum memory. A simple version could function as a repeater, extending the distance covered by quantum networks. Today, the lack of such a device restricts quantum communication to, at most, approximately 100 km in fiber.
Another likely necessity will be the integration of many devices into a small volume, something difficult to do with current sources. Work done by Toshiba Research Europe with electrically driven quantum-dot LEDs points the way toward one solution. R. Mark Stevenson, a senior research scientist at Toshiba, helped create these LEDs, which have quantum dots of very precisely controlled size. Tests have shown that the LEDs emit single pairs of entangled photons. These pairs could form the basis for subsequent quantum processing, with the pairs split up but still connected to one another because of entanglement.

A fully developed commercial entangled LED, with an artist’s rendering of the entangled photon pairs streaming forth. Courtesy of Toshiba Research Europe.
Since the original report on the research appeared in Nature in 2010, work in this area has continued. One goal is to make pairs of emitted photons virtually identical so that they can interact with one another.
“One of the ways that happens is through two-photon interference. The photons have to be indistinguishable in order for successful two-photon interference. We have recently achieved this with light emitted by our entangled LEDs,” Stevenson said.
In work with a similar goal, researchers at the Washington-based National Institute of Standards and Technology have made photons from two different single-photon sources indistinguishable. NIST’s Glenn Solomon and others described their work in a 2011 Physical Review Letters paper. They used filters and other devices to alter the photons’ spectral shapes and other properties. The photons were then so alike that a hybrid quantum network was conceivable, Solomon stated.
Such advances are important to the future of quantum processing in general, said NIST physicist Joshua C. Bienfang, because of the likely nature of future quantum communication networks and computers.
As Bienfang said, “The device that processes information, on which you can implement quantum logic gates, and the device that acts as a memory to store quantum information for later use are probably going to be different machines with different optical properties.”
Making More Money in the Meantime
While working on quantum communication, companies have turned elsewhere for additional revenues. A case in point comes from Geneva’s ID Quantique SA. As with others, its implementation of quantum key distribution requires a random number generator as the basis for cryptography.
The company achieves this by generating, then detecting, a single visible photon in a secure enclosure. A matchbox-size component converts quantum-derived random fluctuations into a string of 1’s and 0’s. Because the device can be mounted on a card in a computer or server, the technology has found a home in many applications far removed from quantum communication.
“Our largest market for this product is the online gaming market, where they need randomness. They need good randomness, for example, to draw winning numbers or to shuffle card decks,” said ID Quantique CEO Grégoire Ribordy.
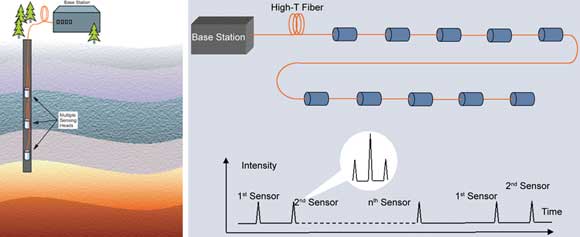
A quantum key distribution system can function as a single-photon interferometer (above right), creating a passive fiber sensor for harsh, borehole environments (above left). Courtesy of MagiQ Technologies.
Another example comes from MagiQ Technologies Inc. of Somerville, Mass. Anton Zavriyev, director of research and development, said the company is engaged in a project for the US Department of Energy. The goal is to develop a passive fiber sensor for harsh environments, such as those found deep within geothermal or oil and gas boreholes.
The entire length of a quantum key distribution system is a distributed single-photon interferometer that can make such measurements, Zavriyev said, adding that it operates with extremely low signal levels and offers other advantages. “By means of optical interferometry, you can be 100 kilometers away from a location and yet know exactly what happens there,” he said.