Compound laser light sources are transforming the possibilities of medical imaging. Selective band and autofluorescence imaging are simple and cost-effective ways for physicians to improve their diagnostic accuracy.
ANDREW GRAVELEY, NATHANIEL GROUP INC.
While halogen, xenon or LED light sources may be sufficient for larger endoscopes, the demand for more extensive imaging through the body is driving endoscopes to smaller sizes. As cameras continue to reduce in size, the diameter of the illumination fiber must also be reduced. In this case, traditional light sources are inferior in their ability to output light through small fibers, while laser light can maintain high output through fibers nearing the size of a strand of hair.
Furthermore, the use of a compound laser light source grants the ability to drastically alter the spectral response through the control of individual lasers without additional changes to the optical system. Such versatility has catalyzed the design and development of endoscopic laser light sources.
Of the many medical applications for endoscopes, cancer diagnosis is among the most prominent. The prognosis of cancer treatment is highly dependent on the stage at which the disease is diagnosed, thus prompting doctors to take proactive measures to find neoplasms before they mature or metastasize. While early stages are significantly easier to treat, they are also more difficult to diagnose. Visible light endoscopy — in which a broad spectrum of white light is used to illuminate the subject — is often limited to diagnosing later stages of carcinogenesis. Unfortunately, simply improving the image quality of current endoscopes is not enough to improve the diagnostic ability; in reality the trouble lies in the properties of the visible light spectrum and how it interacts on a small scale with human tissue. The differences in the altered tissue anatomy of neoplasms are too minimal for effective demarcation of the malignant tissue when examined under white light1,2,3.
Selective band imaging
The issue of distinguishing neoplasms and nonvisible growth is overcome by the use of alternative optical techniques to visualize organs and tissue (Figure 1).
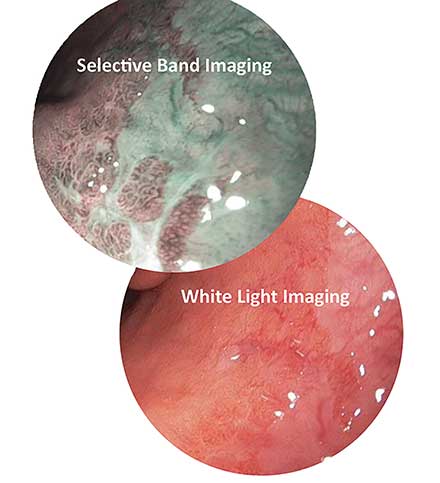
Figure 1. Selective band imaging enhances the visibility of high-grade dysplasia in Barrett’s esophagus. Courtesy of Nathaniel Group.
Carcinogenic regions are known to form dense vasculature to supply the forming tumors with nutrients; one useful technique exploits this characteristic by enhancing the optical contrast of superficial vasculature.
Selective band imaging (SBI) is a method in which tissue is illuminated with two narrow bandwidths of light that correspond to the peaks in the absorption spectra of hemoglobin. This gives the appearance of darkened blood vessels and capillaries as they absorb the bandwidths of light used. The dark vasculature contrasts the lighter, more reflective surrounding tissue, allowing areas such as carcinomas to be revealed. The compound laser Hyperion technology developed by Nathaniel Group Inc. allows users to implement this technique as an optional SBI mode, in addition to the standard white light mode. With this technology, the user can simply select the mode controlled by the firmware, without the use of optical filters on the camera or light source. Using the available controls, the light source can be switched from standard imaging to diagnosis-enhancing SBI. Additional benefits include less power consumption, less heat dissipated by the light source and less heat concentrated in the optical components of the illumination device for equal or greater light output.
In a different approach, use of only near-UV wavelengths is the basis of fluorescent imaging systems that can reveal both visible and nonvisible early stage cancers alike. Fluorescence occurs when a molecule known as a fluorophore is excited by a specific frequency of light before releasing the absorbed energy at a lower wavelength (Figure 2). Fluorescence imaging systems use near-UV light to excite exogenous fluorophores that accumulate in cancerous tissues. The near-UV excitation wavelength must be filtered out, so the image only shows light emitted from the fluorophores. This mechanism, which reveals an image based on a molecular concentration associated with abnormal tissue, is known as exogenous fluorescence diagnosis (EFD).
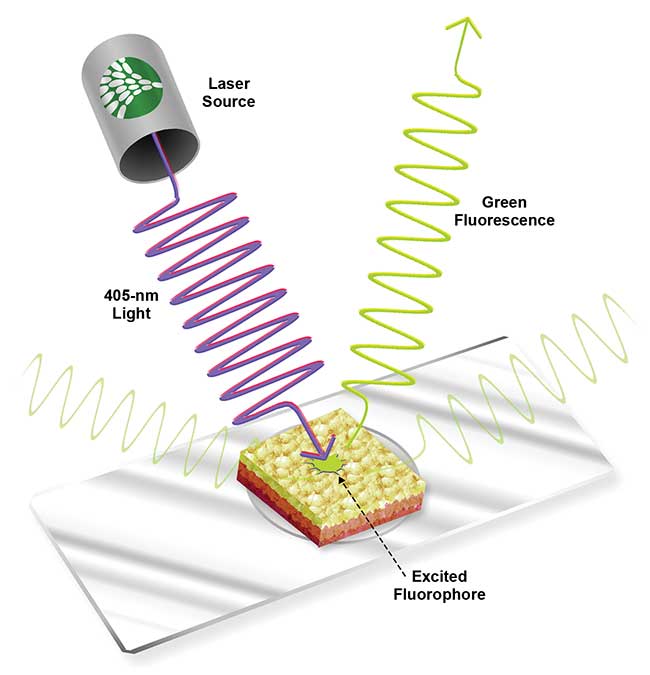
Figure 2. Green fluorescence modeled in tissue with a 405-nm excitation source. Courtesy of Nathaniel Group.
Autofluorescence imaging
Under normal circumstances, tissue walls of the respiratory tract, digestive tract and other organs will fluoresce under near-UV light4,5. Consequently, several molecules found in epithelial tissue have been identified as fluorophores under near-UV light, including collagen, elastin, keratin, NADH and protoporphyrin4,6.
Research by Zhiwei Huang at the National University of Singapore has shown that the primary source of fluorescence in the gastrointestinal wall is the high density of collagen in the submucosal layer, outputting green luminance when excited with a near-UV light source. In contrast, carcinomas in these tissues are known to appear dark in fluorescent imaging, allowing the cancerous cells to be demarcated5. The mechanism for this change is primarily anatomical. The cancer does not significantly alter the concentration of fluorophores, but rather the superficial mucosa layer of the epithelium thickens, thereby dampening the light transmission to and from the fluorescent collagen in the deeper submucosa (Figure 3). Fluorescence in cancerous tissue is further reduced through the light absorption from hemoglobin as the cancer increases localized bloodflow5,3.

Figure 3. This illustration depicts the mechanism of autofluorescence demarcation. Healthy tissue fluoresces from the collagen found in the submucosa, while the enlarged mucosal layer on cancerous tissue reduces the light transmission and thus the fluorescence. Courtesy of Nathaniel Group.
In clinical applications, autofluorescence poses minimal side effects to the patient, while significantly improving the diagnostic sensitivity when compared to white light endoscopy3,6,7,8. In a 2005 study by the Academic Medical Center of the Netherlands, endoscopies were performed on 60 patients with Barrett’s esophagus, a condition that predisposes esophageal cancer. During their procedures, white light endoscopies only revealed 63 percent of all early cancer diagnoses confirmed histologically. However, using autofluorescence imaging (AFI), they were able to catch 91 percent of the total early cancer diagnoses in patients with Barrett’s esophagus. A later 2010 study of 71 patients also reported that AFI at 405 nm provided better diagnostic precision for cancer and dysplasia than white light and other state-of-the-art imaging technologies8 (Figure 4).
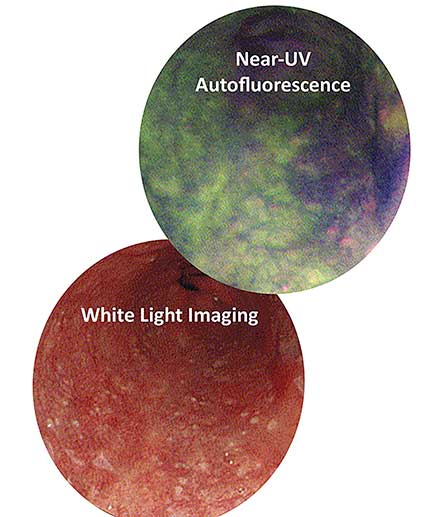
Figure 4. The area in dark purple reveals a suspicious lesion under autofluorescence, while it eludes detection under white light. Biopsy later confirmed the lesion to be precancerous. Courtesy of Nathaniel Group.
A patent-pending technology that utilizes this optimal wavelength for fluorescence and SBI imaging (Figure 4) can be used without additional optical filtration. With so much research in support of autofluorescence, it is surprising that it has not become a standard method for prescreening applicable cancers.
In actuality, the major limitation of AFI is its inability to distinguish precancerous tissue from areas of healthy but inflamed tissue, leading to false positive diagnoses7. While AFI’s high sensitivity and low specificity is acceptable from a safety standpoint, false positive diagnoses will lead to unnecessary costs in time and lab tests, as well as distress for the patient. Fortunately, with a simple technique, AFI can be supplemented with the use of SBI to reduce the rate of false positives. In a study in which 20 patients with Barrett’s esophagus were screened for high-grade dysplasia, physicians checked the results of AFI with SBI, where vasculature patterns were a distinguishing criteria7. This reduced the false positive rate from 40 to 10 percent; a majority of the remaining false positives were low-grade dysplasia7.
For a physician using the Hyperion video-endoscopic system, this comparison only requires the user to select the desired wavelength to examine a suspicious lesion using autofluorescence and then SBI.
A promising drug for fluorescence
To expand the fluorescence capabilities of medical diagnostics, many pharmaceuticals are on the market and under development to improve the detection of disease. Cysview, Hexvix, Levulan and Metvix are some of the medications approved worldwide for the use of EFD9,10. These are an assortment of drugs derived from the endogenous molecule, 5-aminolevulinic acid (ALA). As found in normal cellular processes, ALA is a metabolic rate-limiting precursor to protoporphyrin IX (PpIX), which is fluorescent under near-UV light4.
After prodrug-classified ALA is administered either orally or topically, within a few hours PpIX accumulates in carcinomas in significantly higher concentration relative to surrounding tissue9,11. This accumulation allows the fluorescent positive identification of carcinomas. A major benefit of ALA over current drug therapies is its ability to metabolize within 48 hours, reducing the time a patient must avoid light exposure11.
In nonmuscle invasive bladder cancer, an endoscopic surgical procedure known as a transurethral resection is a preferred treatment, as it is minimally invasive; it has, however, been shown to be less than reliable. As bladder cancer has an indistinct appearance under white light, portions of cancerous tissue are often overlooked by surgeons, leading to high recurrence rates10. However, in conjunction with ALA fluorescence diagnosis, the visual aid of tissue fluorescence during transurethral resection has been shown to reduce recurrence rates by 20 percent12.
Similar improvements in other endoscopic procedures have been reported when implementing fluorescence diagnosis — cervical premalignancies can be better identified and more accurately diagnosed, as tissue for biopsy can be better selected by fluorescence demarcation10.
Choosing between exogenous and autofluorescence
While pharmaceuticals can be used for both diagnostics and treatment, it may not always be the best option when diagnosis is the primary concern. AFI- and ALA-induced EFD both have very high sensitivity, with slight compromises in specificity5,7,13,14.
Considering the added cost of a pharmaceutical, prolonged procedures and side effects that are inherent with the use of EFD, autofluorescence may be preferable for cancer screening. While it was previously believed that autofluorescence illumination was inadequate for camera sensors, recent advances in endoscopic technology, such as the improved light output of the Hyperion, have made exogenous fluorophores no longer necessary for quality imaging.
Exploiting photosensitive side effects
Fluorescent hematoporphyrin was the first ever chemical used for photodynamic therapy (PDT) when it was discovered to concentrate in cancerous tissue in the mouth15. This prompted investigation into the use of this fluorophore for identifying cancer. It was subsequently discovered that this chemical has photosensitizing effects. More recently, similar porphyrinoids such as Photofrin have been developed to selectively accumulate at phototoxic concentrations in the targeted carcinomas, without posing a risk to healthy tissue. Such advantages have made PDT increasingly practical as a targeted treatment for certain cancers and other diseases. Various drugs of this class have been approved for clinical use worldwide for PDT, and more are in development. Photofrin, though currently the most popular drug for PDT, has the tradeoffs of requiring a high dose of light, a slow onset of effectiveness, and a clearance time of over four weeks for which the patient must avoid light. Although it is an effective, and especially preferred treatment for some cases, the improvements of newer photosensitizers will make PDT more suitable for patients.

Table 1. Included here are drugs either currently approved or undergoing clinical trials for the use of photodynamic diagnosis, compared by important parameters of their effectiveness and side effects. The irradiation is the optimal light source wavelength to activate the drug, J/cm2 is the energy of the light, onset is the time the drug must be administered before therapy, and clearance is the time it takes to be removed from the patient’s system, for which the patient must avoid light exposure. Courtesy of Nathaniel Group.
Implementing new technologies
A compound endoscopic laser light source capable of multiple imaging modalities with improved power output — all without the use of optical filters — empowers users to select the imaging mode, whether it is near-UV fluorescence, SBI or standard white light. The ability to easily switch between complementary SBI and fluorescence imaging modalities will soon allow doctors to get more accurate results from endoscopic cancer screenings and optimize cancer prevention. With the development of new drugs for PDT (Table 1), the same light sources used for diagnostic imaging will even be adapted for future cancer treatment thanks to the versatility of laser light.
Meet the author
Andrew Graveley interned at Nathaniel Group while attending the University of Rochester. He graduated with a Bachelor of Science degree in biomedical engineering and is pursuing a career in medical device innovation based in Nashville, Tenn.; email: [email protected].
References
1. J. De Leeuw and W.D. Neugebauer, et al. (2009). Fluorescence detection and diagnosis of non-melanoma skin cancer at an early stage. Lasers Surg Med, Vol. 41, Issue 2, pp. 96-103.
2. H. Messmann and E. Endlicher, et al. (Oct. 2002). Fluorescence endoscopy and photodynamic therapy. Dig Liver Dis, Vol. 34, Issue 10, pp. 754–761.
3. J. Haringsma and G. Tytgat, et al. (May 2001). Autofluorescence endoscopy: Feasibility of detection of GI neoplasms unapparent to white light endoscopy with an evolving technology. Gastrointest Endosc, Vol. 53, Issue 6, pp. 642–650.
4. D. Campbell and E. Gudgin-Dickson (1996). Detection of early stages of carcinogenesis in adenomas of murine lung by 5-aminolevulinic acid-induced protoporphyrin IX fluorescence. Photochem Photobiol, Vol. 64, pp. 676-682.
5. Z. Huang and W. Zheng, et al. (2004). Laser-induced autofluorescence microscopy of normal and tumor human colonic tissue. Int J Oncol, Vol. 24, Issue 1, pp. 59-63.
6. D.C.G. De Veld and M.J.H. Witjes, et al. (2005). The status of in vivo autofluorescence spectroscopy and imaging for oral oncology. Oral Oncol, Vol. 41, Issue 2, pp. 117-131.
7. M. Kara and F. Peters, et al. (August 2006). Endoscopic video-autofluorescence imaging followed by narrow band imaging for detecting early neoplasia in Barrett’s esophagus. Gastrointest Endosc, Vol. 64, Issue 2, pp. 176–185.
8. D. Roblyer (December 2010). Comparison of multispectral wide-field optical imaging modalities to maximize image contrast for objective discrimination of oral neoplasia. J Biomed Opt, Vol. 15, Issue 6.
9. U.S. Food and Drug Administration (May 2010). Drugs@FDA. FDA Approved Drug Products.
10. S. Yano and S. Hirohara, et al. (March 2011). Current states and future views in photodynamic therapy. J Photochem Photobiol, Vol. 12, Issue 1, pp. 46–67.
11. H.S. Freeman and A.B. Ormond (2013). Dye sensitizers for photodynamic therapy. Materials, Vol. 6, Issue 3, pp. 817-840.
12. T. Filbeck and U. Pichlmeier, et al. (July 2002). Clinically relevant improvement of recurrence-free survival with 5-aminolevulinic acid induced fluorescence diagnosis in patients with superficial bladder tumors. J Urol, Vol. 168, Issue 1, pp. 67– 71.
13. E. Endlicher and R. Knuechel, et al. (March 2001). Endoscopic fluorescence detection of low and high grade dysplasia in Barrett’s oesophagus using systemic or local 5-aminolaevulinic acid sensitisation. Gut, Vol. 48, Issue 3, pp. 314–319.
14. R. DaCosta and B. Wilson, et al. (2006). Spectroscopy and fluorescence in esophageal diseases. Best Pract Res Clin Gastroenterol, Vol. 20, Issue 1, pp. 41-57.
15. J.R. Leonard and W.L. Beck (March 1971). Hematoporphyrin fluorescence: An aid in diagnosis of malignant neoplasms. Laryngoscope, Vol. 86, Issue 5, p. 661.