When it comes to attention-to-detail, few industries compare with the medical sector, where flawless products are required. Here, the most precise devices can be life-changing — or even life-saving — for patients.
Ultraprecision manufacturing and optics unite two sophisticated technologies that are essential for a modern world that demands exact and meticulous results. From spacecraft components, next-generation displays and electronic devices to low-cost photovoltaic cells, defense and security technologies, ultraprecision machining is the catalyst behind much of today’s frontline products.
The pioneers driving this area of industrial fastidiousness come from many different sectors. Photonics approaches are largely dominated by laser machining, but there are many other methods that can sometimes be an alternative or, as in an emerging trend, used alongside each other for superior results.
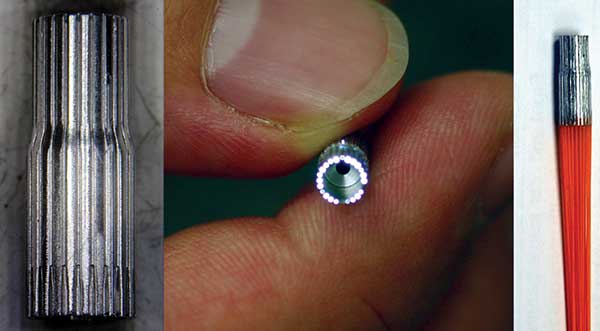
Micromachined fiber guide sleeve for earscanner. Optical fibers become embedded into the axial slots of the guide sleeve (left). Completed fiber guide with lighting fiber ends (right). Courtesy of Kugler GmbH.
Excluding laser micromachining of the circuitry associated with medical devices, the main applications include creating
stents for coronary, peripheral and neurological uses; cutting holes and other features in disposables, such as catheters and infusion/fluid delivery devices; and creating permanent counterfeit-proof marks without surface damage in plastic products, glass syringes and vials.
“Laser micromachining offers the potential for removal or transformation of material with incredible spatial selectivity and three-dimensional flexibility. It is therefore a superior tool for creating small features, and features and edges with smooth surfaces,” said Joris van Nunen, product line manager for Industrial Ultrafast Lasers at Coherent Inc. “It’s also useful for processing very thin materials, and materials that are delicate or thermally sensitive.”

Demonstrating fluid system mold for different lab on-a-chip functions. This is a feasibility study with several structures and geometry elements typical for biochemical fluid systems. Courtesy of Kugler GmbH.
Cool cutting
Whether cutting with a laser or a diamond mill, the heat that is generated as a byproduct should be minimized. The goal is to achieve cold or athermal ablation — removing material with an absolute minimum of peripheral heating, which could manifest as burrs, slag or recast material. Incidentally, this is a common goal in industries such as microelectronics and solar, meaning the manufacturing progression is similarly mirrored.
“This heat-affected zone can be minimized by using shorter wavelengths and/or shorter pulsewidths,” said Frank Gaebler, marketing director for materials processing at Coherent Inc. “For this reason, a few years ago, we saw fast growth in the use of excimers and visible nanosecond DPSS [diode-pumped solid-state] lasers; subsequently, there was a move toward ultraviolet DPSS lasers.”
The next big development came in the form of industrial ultrafast lasers, which produce an even smaller heat-affected zone (HAZ) than nanosecond infrared or visible lasers. Here, femtosecond lasers almost universally deliver superior surface and edge quality compared with any previous industrial laser type.

Mold insert for microfluidic components. Courtesy of Kugler GmbH.
“At first most of these operated in the picosecond regime, but today laser manufacturers now also offer femtosecond performance in industrial-grade platforms,” Gaebler said. “The use of ultrafast laser pulses enables cutting of novel hole shapes and modalities in catheters, for example, and allows marking inside glass products, such as syringe bodies, with no impact on the surface, material integrity or the contents.”
For any production line, process optimization is a challenge. In some cases this can be achieved quickly — a single day — but for some medical precision manufacturing applications, optimization can take a month or more.
As laser pulse widths shorten and surface finish becomes just as critical as throughput and process economics, optimizing the process can mean the difference between success or failure.

Pyramid microstructure for multiwell plate functionalization. This structure was created by diamond machining on the tips of a finger array in a mold for polycarbonate multiwell plates used in medical analytics. In combination with a superimposed nanostructure due to a special CVD-coating of the microstructured mold fingers, the bottoms of molded wells show the lotus effect for aqueous samples. Courtesy of Kugler GmbH.
When it comes to “routine” machining applications using a carbon dioxide laser, for example, process optimization can typically be completed in around a day in a laboratory or at the customer site. Even when using state-of-the-art nanosecond lasers, process optimization rarely takes longer than two days.
“But picosecond laser processes often require a whole week of iterative test runs and improvement,” Gaebler said. “And with femtosecond lasers, some medical precision manufacturing applications can entail a month or more of careful optimization.”
Ultrafast lasers pose another problem: In the past, the reliability of industrial femtosecond, and even picosecond, lasers was not optimal, often compromising 24/7 applications. What’s more, these lasers were only available for a premium price, compared with nanosecond lasers.

Micro-4 Machining from Loxham Precision Ltd., a ‘micro-factory’ precision machining center (Centre for Innovative Manufacturing in Ultra Precision). Courtesy of the Centre for Innovative Manufacturing in Ultra Precision and Cranfield University.
While early adopters were willing to tolerate these issues, laser manufacturers knew they had to address the issues of
process costs and laser reliability before the wider market would welcome the devices.
“In the last five years, we have seen tremendous advances in laser design, manufacturing and testing, and particularly the rigorous use of HALT/HASS testing practices and tools, long used in other nonphotonic industries,” van Nunen said. “The result is a new generation of ultrafast lasers, such as the Coherent RAPID NX and Monaco family, which provide the same levels of reliability and lifetime as users routinely expect from other electronic instrumentation.”
Highly accelerated life test (HALT) and highly accelerated stress screening (HASS) are methods of fast-tracking product reliability that are commonly used by the electronics and computer industries as well as by the military.
The hope is that as automated laser assembly and economies of scale help to push down the cost of femtosecond lasers, combined with levels of production throughput, high uptime and reduced cost of ownership, widespread adoption will be seen in many areas of medical manufacturing.
Molds, microfluidics and medical disposables
Many medical devices such as optical molds, lenses and fibers are machined using nonlaser-based methods such as milling and diamond turning. Medical technologies offer a substantial market for machine tool engineering, which is largely addressed by a number of well-known machine tool manufacturers in the south of Germany, including Kugler GmbH based in Salem, Germany.
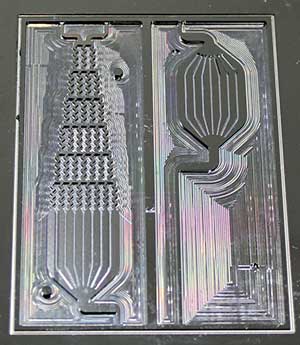
Detail of microfluidic mold insert. Left half shows a mixer and reaction area for two fluids, while the right half depicts an extended flat chamber for interaction of fluid constituents with light. Courtesy of Kugler GmbH.
“The most important application of precision manufacturing by high precision milling and micromilling in medical technology consists in the manufacture of mold inserts,” said Klaus Baier, R&D project manager at Kugler.
Optical molds produce some of the smallest lenses and microlens arrays for optical instruments in medical analytics and diagnostics. Molds are also needed for large volume production of items such as lab-on-a-chip and microfluidic systems; multiwell and microplates; cell and tissue culture flasks; and products for blood, urine and specimen collection, which are increasingly used as medical disposables.
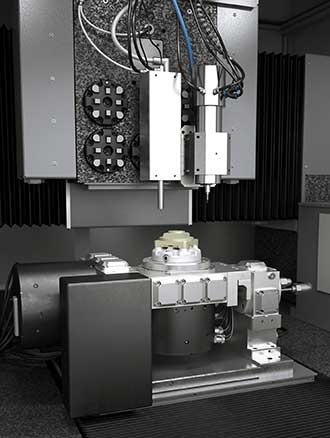
The Kugler MICROGANTRY nano3/5X is a high precision, CNC-controlled 3- to 5-axis machining center with aerostatic bearings, which is specially designed and optimized for the demands of micromachining and microstructuring with the use of microcutting and/or laser technology. The image shows a view into the machining space. The lower Y-axis slide carries a turn and swivel unit with the workpiece. It is spanned by the XZ-axes gantry where a spindle for micromilling and a co-linear touch probe measuring system are attached to the Z-slide. Courtesy of Kugler GmbH.
“Micromilling and microdrilling can be used for the direct preparation of PMMA [polymethyl methacrylate] fibers with diameters less than 500 microns and fiber components which are applied in sensor systems for the minimal invasive blood glucose measurement and in-ear scanners,” Baier said.
The next frontier in medicine will take advantage of microsystems, which play a key role for in-vitro diagnostics and personalized medicine and health care. Implantable drug delivery systems and artificial organs that are currently under development rely on MEMS (microelectromechanical systems) and MOEMS (micro-optoelectromechanical systems) that are products of ultra-precise manufacturing.
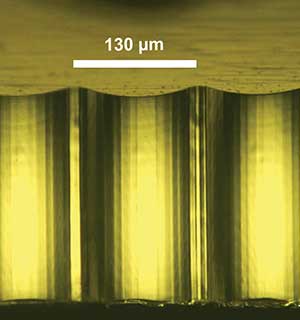
A microscopic picture of a cylindrical micromirror array from diamond shaping. These mirrors are the functional areas of a mold for polymethyl methacrylate parts used in opto-electronic devices with up to 16 parallel waveguides for decoupling the light beams into free space toward the detection chamber with marked cancer cells. Courtesy of Kugler GmbH.
“Machining techniques including diamond turning/shaping and micromilling are a precondition for the manufacture of all kind[s] of microsystems which are indeed on the cusp to revolutionize medical engineering,” Baier said.
Best of both
Competition is fierce in the manufacturing industry and champions of one approach may have once viewed each other as rivals. But things are changing, and an emerging trend is increasingly transforming competitors into collaborators.
“An interesting new market trend we are seeing at Coherent is increasing interest in lasers from companies specializing in various classical tools, such as EDM (electron discharge machining),” Gaebler said.
“EDM has long been used for drilling small holes and etching features, but is limited to metals or substrates precoated with metals. So lasers, and particularly femtosecond lasers, allow these tool builders to equip their systems with a material-neutral tool that provides 3D machining capabilities as a complement or alternative to their EDM technology.”
Researchers at the U.K.’s Centre for Innovative Manufacturing in Ultra Precision based at Cranfield University in Bedfordshire predict the future of innovative manufacturing lies in hybrid machining centers that combine laser with ion beam figuring and plasma with beam figuring.

CAD drawing of the 1.4 m-wide Roll-to-Roll research platform under construction at Cranfield University (Centre for Innovative Manufacturing in Ultra Precision). Courtesy of the Centre for Innovative Manufacturing in Ultra Precision and Cranfield University.
The result is an enhanced surface finish down to subnanometer levels or to 10 nm featuring faster than possible in conventional single processing centers.
“The development of miniature ultra-precision machining centers such as the Loxham Precision [based in Cranfield, U.K.] Micro-4 permit high levels of machining precision at higher volumes than could previously be achieved,” said Martin O’Hara, national strategy manager for Ultra Precision at the Engineering and Physical Sciences Research Council-funded center.
“By using automated loading/unloading, maintaining the machining environment away from the user access and reducing overall moving mass to enable single-phase operation, parts can now be produced with submicron precision in almost any location,” he added.
This enables customized micro-factory manufacturing to be located in retail environments for optical glass manufacture, for example, and clinical environments for ocular implants fabricated to patient requirements.
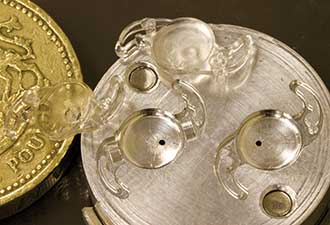
An ocular implant with a micro-fluidic dosing system that eliminates the need for post-operative medicine and/or targets the drug directly to the implant. Courtesy of the Centre for Innovative. Manufacturing in Ultra Precision and Cranfield University.
In another emerging trend, 3D printing offers some enticing benefits for creating reproducible and made-to-measure products for medical applications. But for all of its advantages, 3D printing cannot compete with laser and other traditional processes in terms of speed and cost of operation.v
“At the moment the methods of 3D printing do not yet yield the dimensional accuracy needed for all the parts described above,” Kugler’s Baier said. “Furthermore not all the biocompatible materials are available for 3D printing.”
Again, the future seems to be to integrate the techniques. Experts at Coherent believe that combining novel laser-based additive manufacturing methods with the convenience of 3D printing concepts holds a lot of promise and potential market growth.
An emerging real world example of this involves metal sintering of titanium, which allows for bone (replacement) implants, for example, to replace a jawbone damaged in an automobile accident or removed because of cancer.
“3D scanning followed by 3D printing with a fiber laser enables creation of a perfect replica replacement that is unique and patient-specific,” van Nunen said. “This is a great example of how precision laser machining can improve, rather than simply replace, some existing manufacturing methods.”