Marie Freebody, Contributing Editor
From brain mapping to breaking the laws of physics, optical microscopy has made huge leaps forward in recent years. But as new dimensions open up, it’s clear the real work has only just begun.
Last year yielded a bumper crop of Nobel Prizes for microscopy innovations. Thanks to advances in optical components and the labeling specificity of fluorescent probes, scientists now have a significantly more powerful tool, offering glimpses deeper than ever into the molecules that make life tick.
It was long believed that optical microscopy would never obtain resolution better than half the wavelength of light. To most scientists, this was an accepted and logical fact. But defying the laws of physics is just the kind of research that pushes science into the realm of science fiction – and earns you a Nobel Prize.
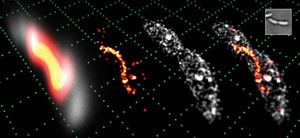
3-D perspective images of a predivisional bacterium taken by the double-helix microscope. Photo courtesy of Matthew Lew, Moerner Lab, Stanford University.
Professors Dr. Eric Betzig, Dr. Stefan Hell and Dr. W.E. Moerner were each awarded the 2014 Nobel Prize in chemistry for landmark work in which they circumvented the diffraction limit of light and enabled optical microscopy to be carried out in the nanodimension.
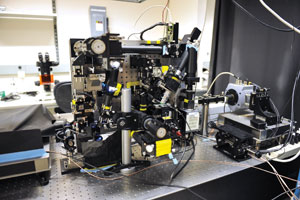
Superresolution microscopes increasingly are adopted for groundbreaking research. Photo courtesy of Matt Staley, Janella Research Campus.
Betzig is a group leader at the Janelia Research Campus at the Howard Hughes Medical Institute in Maryland; Hell is a director at the Max Planck Institute for Biophysical Chemistry in Germany; and Moerner is the principal investigator at the Moerner Lab at Stanford University
in California.
Their work brought about the birth of superresolution microscopy, enabling fine structural details of around 20 to 50 nm – such as cytoskeletons and arrangements of proteins in macromolecular complexes – to be resolved.
By unlocking the nanoworld, scientists can gain a better understanding of the brain, certain diseases and even the embryonic cells where life begins.
“The molecules that drive the living machinery within our cells are simply too small to be resolved from one another using traditional microscopes,” said
Dr. Matthew Lew, who received his PhD working under Moerner at Stanford. “Scientists need noninvasive tools that can study life at the nanoscale in order to, for example, figure out what causes cells to malfunction and become cancerous, or to study how viruses and bacteria invade our cells so that drugs can be developed against them.”
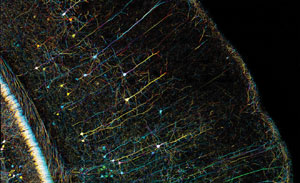
Confocal microscopy of mouse brain, cortex. Photo courtesy of Zeiss and Yi Zuo of the Molecular, Cell and Developmental Biology (MCDB) Department at the University of California, Santa Cruz.
While existing electron microscopes certainly offer high resolving powers, these instruments also require massive preparation. They are limited in multiparameter staining and can be too harsh for use on living cells.
On the other hand, optical technology provides a noninvasive alternative and, with recent advancements, optical microscopes increasingly are being adopted for minute work. One of the biggest advances has come not from a better objective lens or light source, but from the game-changing development of fluorescent proteins.
Their discovery revolutionized the way in which biological cells could be imaged and allowed staining even in live animals. The work earned their developers –
Dr. Osamu Shimomura at the Marine Biological Laboratory and Boston University School of Medicine; Dr. Martin Chalfie at Columbia University in New York; and Dr. Roger Tsien at the Howard Hughes Medical Center – the 2008 Nobel Prize in chemistry.
While microscopy hardware developments may be less revolutionary and more incremental in nature, they are significantly enhancing the standard of microscopy research, as well as opening up new applications.
“Fluorescence microscopy in particular
has benefited from the availability of affordable solid-state lasers at a plethora of wavelengths that can excite a variety of fluorescent probes, high-quality spectral filters that can separate laser light from fluorescent light with high efficiency and minimal aberrations, and the refinement
of electron multiplying CCDs and scientific CMOS cameras that are fast and ultrasensitive,” Lew said.
Microscopy also benefits from advances in today’s sample stages, which now offer nanometer-movement precision and stability, making superresolution experiments more robust.
International distributor Meadowlark Optics Inc. of Frederick, Colo., has released high-efficiency spatial light modulators (Meadowlark XY Phase series) that not only add 3-D imaging capabilities to microscopes, but also allow researchers to see deeper inside cells.
Olympus Corp., a Tokyo-based manufacturer of optical and digital equipment
for the health care and consumer elect-ronics sectors, now manufactures a
high-numerical-aperture objective lens (PLAPON 60X O) that is supercorrected for chromatic aberrations, increasing the accuracy of multicolor fluorescence microscopy.
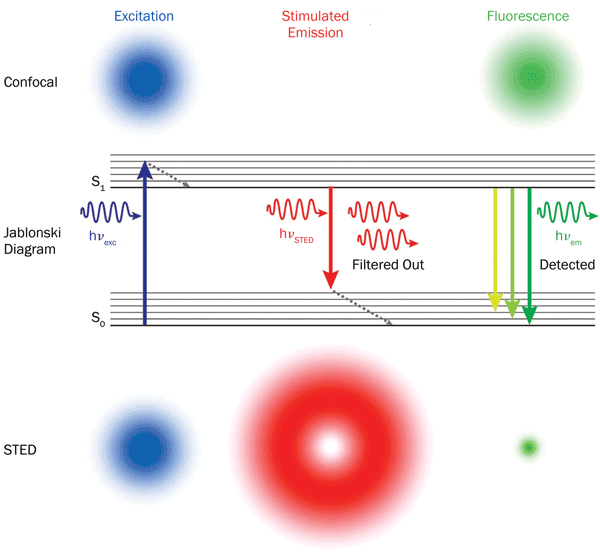
STED photophysics: Fluorophores are excited to a higher energy level (S1) everywhere inside the excitation laser spot (blue). In stimulated emission, a photon triggers a fluorophore in the S1 state to emit a photon with the same wavelength and then relax to the
ground state. Depletion of the excited state therefore silences the
fluorophores where STED light (red) dominates. Photo courtesy of Leica Microsystems.
As far as classical lenses are concerned, senior scientist Dr. Rolf Borlinghaus of Leica Microsystems CMS GmbH of Mannheim, Germany, and science writer Dr. Christoph Greb of Leica Microsystems GmbH in Wetzlar, Germany, believe that objective lenses are pretty much at their limits.
To keep up with the highly specific needs of today’s cutting-edge research, lenses often must be manufactured bespoke. These lenses offer extreme performance in the required optical parameter but may have limited applicability for other purposes.
“One example is the Leica motCORR lens with extreme long working distance, very high aperture (i.e., high resolution) and large field of view,” Borlinghaus said. “Other objectives, for example, were optimized for live-cell imaging at 37 °C and are perfectly fitting the refractive index of living cells or tissue (glycerol and water immersion).”
“The reason for limiting some of the parameters is, in many cases, not the impossibility to make universal lenses, but the complexity and subsequently the cost that would come with all-purpose superperformance lenses,” Greb said.
At Carl Zeiss Microscopy GmbH in Jena, Germany, component developers note that some of the major trends include improving imaging resolution, sensitivity and speed. But perhaps the biggest demand is for 3-D imaging of living cells and tissue at high resolutions.
“Our superresolution system Elyra now is capable of PALM (photoactivated localization microscopy) imaging in 3-D,” said Dr. Klaus Weisshart, product manager for superresolution microscopy at Zeiss. “There are three areas where Elyra systems with PALM [have] already made an impact: particle tracking, as PALM allows scientists to view hundreds of molecules at a time; cluster analysis to study the distribution and aggregation of single molecules; and classical superresolution imaging of subcellular complexes and macromolecules, with the potential to reveal molecule interactions and localizations.”
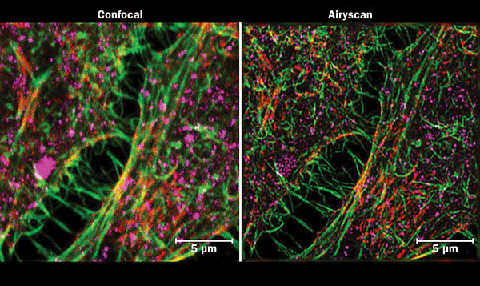
Conventional confocal microscopy compared to Airy scanning of HeLa cells. Matt Staley, Janelia Research Campus
Zeiss and S. Traikov, Biotech Zentrum TU Dresden, Germany
The company’s recently launched Airy-scan detector has brought superresolution-like improvements to its range of confocal laser scanning microscopes, and new objectives now support tissue-clearing methods for Lightsheet Z.1. There are also high hopes that upcoming technologies such as lattice light-sheet microscopy will allow for gentle live imaging of cells in 3-D at unprecedented speed and resolution.
Left: The Elyra PS.1 3-D superresolution system with LSM 880 from Zeiss. Center: Leica’s SR GSD 3D superresolution system enables 3-D localization microscopy. Right: Leica’s TCS SP8 STED 3X superresolution system reveals the full spectrum of life in 3-D, quickly and directly.
Microscopy blazes a trail
Optical microscopy is widely used and, in recent years, has led to a host of pioneering research, such as the tracking of proteins involved in Parkinson’s, Alzheimer’s and Huntington’s diseases; visualizing the individual proteins in fertilized eggs as these divide into embryos; and unlocking the secrets of how the brain operates:
• The first live video of synaptic processes was recorded in 2008 by Dr. Silvio Rizzoli at the University of Göttingen in Germany using STED microscopy. Since then, Rizzoli has reconstructed the first scientifically accurate 3-D model of a synapse.
• A better understanding of one source of cell health was achieved in 2008 by examining the nuclear pore complex using the Leica SR GSD wide-field superresolution system.
• Knowledge of the human immune response against viruses was extended by Dr. Michaela Weber of Philipp University of Marburg, Germany, and colleagues in 2013 by turning the powers of ground-state depletion imaging (GSDIM) on the antiviral proteins that interact with a virus.
• While astronaut Koichi Wakata of the Japan Aerospace Exploration Agency stayed on the International Space Station in 2014, he studied the effect of microgravity on bone density and on plant growth with an inverted research microscope by Leica Microsystems (the Leica DMI6000 B).
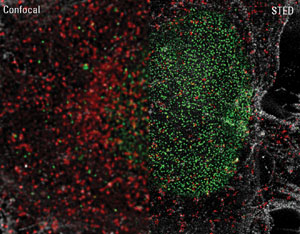
STED image of triple immunostaining in HeLa cells. Green: NUP153-Alexa 532, red: Clathrin-TMR,
white: Actin-Alexa 488. Photo courtesy of Leica Microsystems.
• Mapping gene activity in the brain using Leica Microsystems’ Laser Microdissection devices is helping researchers at the Allen Institute for Brain Science in Seattle to decipher some of the complexities of the brain.
• Dr. May-Britt Moser and Dr. Edvard Moser, 2014 Nobel laureates at the Kavli Institute in Trondheim, Norway, use a multitude of Zeiss microscopes, including Stemi 2000, confocal light-sheet microscopy systems and wide-field fluorescence instruments, to better understand how the brain maps the world around us.
The real work begins
Despite the impressive science that already is under way, Dr. Wesley Legant, a postdoctoral student working with Betzig at the Janelia Research Campus, believes that we are seeing just the tip of the iceberg.
“I think that as more biologists start using superresolution techniques, we’ll see even more applications,” he said. “Until now, a lot of the work has been on technique development. Only recently are the useful applications really starting to come out.”
But challenges remain, and there is still significant room for component improvement, as well as a need to better control fluorescent molecules.
“For instance, with the increasing interest and adoption of 3-D superresolution microscopy, new objective lenses that have aberration corrections over an entire 3-D volume, not just corrections in their native focal plane, will be extremely useful,” Lew said.
Further developments will also hinge upon improving brightness and blinking rates of fluorescent molecules, as well as the algorithms for analyzing resulting images, which many point out are just as important as developing the optics of the microscopes themselves.
“Our close partnerships with leading scientists enable us to identify research trends and emerging technologies quite early, so [we] can expect more
exciting innovations in the future,” said Dr. Johannes Amon, who is responsible for communications at Zeiss Microscopy.