In March, the first article in this two-part series looked at the potential of photoacoustic technologies to revolutionize cancer detection. This second part will examine uses in diagnosing blood disorders, monitoring diabetes and more.
Advances in optoacoustic/photoacoustic (PA) technologies will impact biological discoveries and also clinical applications as well as more traditional applications such as spectroscopy and sensing for industrial, environmental and other applications, according to professor Vasilis Ntziachristos of Technical University Munich and the Helmholtz Center Munich.
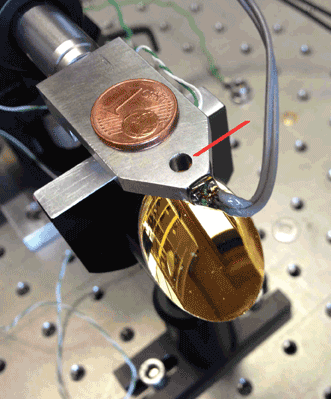
A close-up photograph of a photoacoustic measuring cell. The hole is the open resonance cell; the microphone is contacted sideways. Below is the mirror that focuses the IR beam into the cell. Courtesy of Dr. Werner Mäntele, Johann Wolfgang Goethe University.
“There is now a field of users of optoacoustic technologies which do not come from the traditional physics and engineering laboratories but are attracted to the technology because of its performance in sensing and imaging biological specimen and animals,” Ntziachristos pointed out, adding that he expects similar growth in microscopy/mesoscopy, too. “Clearly, however, this is a technology with significant clinical value and is expected to shape the landscape of optical clinical imaging by enabling – for the first time – three-dimensional high-resolution imaging of optical contrast in diagnostics and theranostics.”
Resolution is a key advantage. “Photoacoustics … can provide us with an imaging modality that (a) has good contrast and scalable resolution (since it relies on ultrasonic detection) and (b) can provide functional information related to blood oxygenation,” said Dr. Michael Kolios of Ryerson University in Toronto. “Scalable resolution means that superficial structures can be imaged with very high resolution, and deeper ones with typical clinical ultrasound detection resolution.

Ryerson’s microfluidic device attached to an optical microscope: An ultrasound transducer is attached at the top, and a laser is focused into the device by the optical objective from below. Cells are streamed through the target area, where they are irradiated with the laser, and the resulting photoacoustic signals are recorded by the ultrasound transducer. Courtesy of Dr. Michael Kolios, Ryerson University.
“One can either rely on endogenous chromophores for contrast (such as blood vessels and red blood cells) or use nano-scale contrast agents (such as plasmonic nanoparticles) conjugated so as to bind to the target of interest.
“In the former application (for the vasculature), this is one of the few imaging modalities that is exquisitely sensitive to the oxygenation status of blood and therefore provides functional information about the tissues. In the latter application, because of the large photoacoustic signals that the nanoscale contrast agents generate, photoacoustic imaging can offer in principle very good sensitivity (a bit like positron emission tomography, but with real-time imaging).

A correlation between “measured” invasive glucose testing (with test sticks on blood drops) and “predicted” noninvasive testing (from IR photoacoustic data). The RMSECV (root mean square error of cross validation) is at about 12 mg/dL – not as good as values obtained in clinics, but better than most test-strip systems. The black lines are the Clarke error grid: All systems that are to be applied for patients and in clinics should show >90 percent of the data points within the grid. Courtesy of Dr. Werner Mäntele, Johann Wolfgang Goethe University.
“Since as soon as these acoustic waves are generated, the physics of ultrasound propagation dictates the imaging approach used, many methods developed for ultrasound can be adopted to photoacoustic imaging, including quantitative ultrasound approaches.”
The first article in this series focused mainly on cancer-related applications, but cancer isn’t the only disease that can be caught with photoacoustics.
Diagnosing blood disorders
In an October news story (“Blood cells’ songs reveal their secrets,” BioScan), BioPhotonics covered a study from Kolios and colleagues in which they had discovered that red blood cells struck with laser light generate high-frequency sound waves, and that these could help them differentiate normal cells from abnormal ones to diagnose blood disorders such as malaria or sickle-cell anemia. The research appeared in Biophysical Journal (doi: 10.1016/j.bpj.2013.05.037).
“I predicted several years ago that my future research direction would be ‘bloody,’ even though my lab [members] had never dealt with blood before,” Kolios said. “Our lab is now investigating methods by which you can detect circulating tumor cells using microfluidics, red-blood-cell aggregation using quantitative ultrasound spectroscopy of the photoacoustic signals to assess the quality of stored blood, and the development of theranostic agents (agents that can be used in both an imaging and therapy capacity).
“I am very interested in adapting quantitative ultrasound techniques to analyze photoacoustic signals – i.e., a careful analysis of the frequency content of the photoacoustic signals generated. Our recent work on the analysis of photoacoustic signals of red blood cells using gigahertz ultrasound generated considerable interest.”
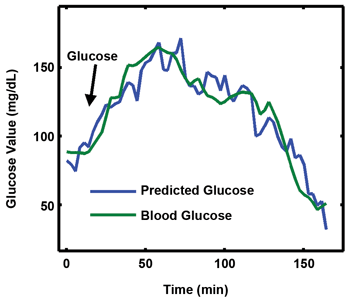
A comparison of time course for “real glucose” measured on blood with test sticks (green line) and “predicted glucose” obtained from the analysis of the IR photoacoustic signals: The modulation of blood glucose was obtained by uptake of 75 g of glucose in 300 mL of water at the beginning of the experiment. Courtesy of Dr. Werner Mäntele, Johann Wolfgang Goethe University.
The researchers are now working to develop a microfluidic device that integrates the laser and probes to flow single cells through a target area. “This would enable measuring thousands of cells in a very short period of time with minimal user involvement,” said Eric Strohm, a graduate student in Kolios’ laboratory.
Diabetes monitoring, diagnosis
In another recent news story (“Photoacoustic spectroscopy takes sting out of glucose testing,” January BioScan), BioPhotonics covered a noninvasive glucose-monitoring method using mid-IR photoacoustic spectroscopy, which could take finger pricks out of the equation for the millions of people living with diabetes; the work was published in Review of Scientific Instruments (doi: 10.1063/1.4816723).
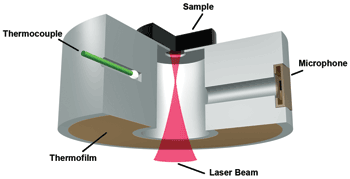
A 3-D drawing of the photoacoustic cell. The vertical cylinder is the absorption cell; the smaller, horizontal one with the microphone at the end is the resonance cylinder. Together they form a Helmholtz cell, open at the lower end for the entry of the IR laser, and closed at the upper end by the skin. Courtesy of Dr. Werner Mäntele, Johann Wolfgang Goethe University.
The lead researcher behind that development is Dr. Werner Mäntele, managing director of the Institute for Biophysics at Johann Wolfgang Goethe University in Frankfurt.
“Photoacoustics always was sort of a niche technology,” Mäntele said, “mainly because the laser wavelengths to excite PA were limited to the UV, VIS and only a few infrared wavelengths. However, with the development of tunable infrared quantum cascade lasers (QCLs), infrared excited photoacoustics is possible.”
Selectively exciting molecular vibration has been an enormous advantage, he added, and the high laser power available from QCLs has given photoacoustics increased sensitivity, which he said will open even more applications in medicine and biochemistry/biotechnology in the coming years.
“Tomographic techniques based on photoacoustics are already in [the] pre-clinical test phase, and quite promising,” Mäntele said. “I could imagine skin analysis, depth profiling and even melanoma analysis of skin by PA techniques very soon. With the chemical selectivity provided by tunable infrared laser excitation, noninvasive analysis techniques for body fluid constituents (such as our noninvasive glucose measurement in skin) become possible.”
The next step is to study the method more closely. “Our focus is on noninvasive glucose measurement in the interstitial layer of skin,” Mäntele said. “Glucose in this layer, approximately 20 to 80 µm below [the] skin surface, is closely correlated to blood glucose and thus the most direct way to determine glucose values for the diabetes patient. We think that we have demonstrated the feasibility of a noninvasive glucose measurement for healthy volunteers and diabetes patients and will now study the practical use of this technique in the day-to-day and person-to-person variation of skin with a small number of volunteers.
“We call this a ‘walk-in study,’ since the measurements will be performed on our laboratory setup. This allows us to constantly improve the technology and yet to perform studies on patients.”
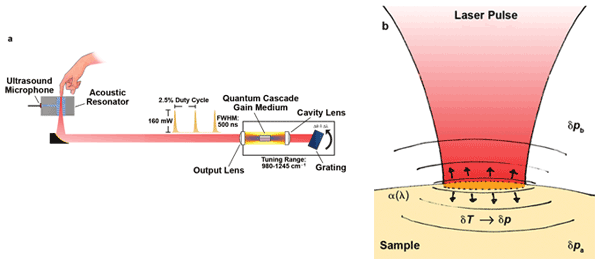
These schematic drawings show a photoacoustic setup (a) and how the photoacoustic effect arises in a sample (b). FWHM = full width half maximum. Courtesy of Dr. Werner Mäntele, Johann Wolfgang Goethe University.
The technique still needs some refinement, he added. “Improvements on the technical side include better QCL lasers, more powerful and with wider tunability, and further improvements on the design of PA cells, based on simulations of cell designs. We will also use alternative techniques for the PA detection, such as photoacoustic deflection spectroscopy and quartz-enhanced photoacoustic spectroscopy.
“Without being [too] optimistic, I think we will have a shoebox-sized, portable prototype of a noninvasive glucose measuring device in two to three years that can already serve in clinical use, diabetes centers, etc. As prices for QCLs will be falling, it requires some years of engineering to produce a handheld device, probably looking like one of the first mobile phones. Our institute will need strong industry partners for both steps.”
Future directions
Translating any advances to clinical applications will take time, of course. “Although it is difficult to make predictions on the exact time frame of clinical dissemination, I strongly believe that the clinical acceptance of optoacoustic/photoacoustic methods is inevitable in the next years, especially in clinical areas already served by optical methods (endoscopy, surgery, dermatology), and areas where ultrasound imaging is employed,” Ntziachristos said.
“If we [focus on] biological imaging, we see today that optoacoustic/photoacoustic technology is a game changer for optical imaging,” he added. “By enabling high-resolution optical imaging beyond the optical microscopy capacity and by utilizing spectral capabilities, the approach brings unprecedented capacity in visualizing anatomical, functional and molecular features in high resolution in the mesoscopic and macroscopic regime.
“Simply put: No other imaging modality can offer such performance and versatility.”
The technology might not become as commonplace in medical clinics as CT and MRI are today, Kolios predicted. “However, for specific applications where imaging is required in the first few centimeters of tissue, then photoacoustic imaging technologies might be the approach of choice, as it can image both structure and function. I can foresee applications for which analysis of blood is done noninvasively by interrogation of an appropriate superficial vessel, such as the radial artery.”
He said he’s happy to see commercial products already available on the market, “both preclinical and clinical imaging systems. Once the technology gets disseminated to end users that need to address biological or clinical questions, then I’m confident the technology will be used in ways we haven’t even imagined.”