Scott York, Photonic Science Ltd.
Shortwave infrared imaging is finding new applications by improving spectroscopy, inspection and more.
A whole new waveband of the electromagnetic spectrum has been opened up for exploitation in the past few years – the shortwave infrared (SWIR), which ranges from the edge of the near-IR region at 900 nm up to 1700 nm and has traditionally been invisible to all detectors. Now, indium gallium arsenide (InGaAs) sensors can image within this waveband for a wide range of applications: Stimulated photoemission silicon devices can allow in-line inspection of their internal structure during manufacture; bruising of fruit can be detected by imaging the subsurface accumulation of water; surveillance applications can benefit from reduced atmospheric scattering due to mist in the SWIR band, and can take advantage of the SWIR-band “nightglow” of a clear night sky; and SWIR-band laser illuminators, invisible to most detectors, can provide high-quality night vision when coupled with a SWIR camera.
So how can we take advantage of this new part of the electromagnetic spectrum? The first obvious use is in spectroscopy. A normal CCD color camera has sensitivity up to about 700 nm, at which point the IR cut filter cuts off the near-IR that would otherwise ruin the color balance of scenes intended to look natural to the human eye. A monochrome CCD, without the IR cut filter, will see up to about 1000 nm. An InGaAs SWIR sensor, on the other hand, has sensitivity from about 900 to 1700 nm and therefore makes an ideal component of a multisensor system for hyperspectral imaging. Cameras are available now with waveband extension up to 2200 nm and even with visible extension down to 400 nm, making a very wide spectral range possible within a single camera.
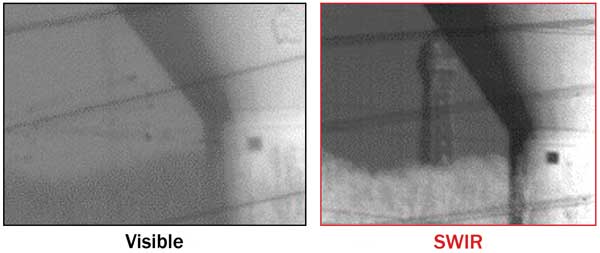
Figure 1. The two images show the same vista, but the right is taken using an InGaAs CACTUS320 SW camera from Sofradir and a visible-SWIR lens with 1.5-m focal length, while the left is taken using visible light. Note that the Eiffel Tower is clearly seen in the SWIR image at 25 km. Photo courtesy of Sofradir and Thales.
A recent spectroscopic application here is in remote detection and identification of chemical spillage and staining. Materials absorb, reflect and even fluoresce in unfamiliar ways in the SWIR spectrum. Characterizing these effects can allow noncontact identification of materials to be made where, previously, physical samples would have to be taken for chemical analysis. In the recent EU-funded Opticlean project supporting the pharmaceutical industry, for example, the spectral signatures of chemical stains under broadband illumination are measured in 120 wavelength bands throughout the SWIR spectrum, using filters and an interferometer to isolate the individual wavelength contributions. Comparing this signature with those of precharacterized samples allows the nature and extent of the staining to be identified.
Conventional thermal cameras, operating in the wavebands of 3 to 5 and 8 to 12 µm, are well known for their ability to detect body heat. SWIR cameras detect temperature changes too, but at higher temperatures (about 100 ºC and above). This makes them a good choice for remote temperature mapping in industrial conditions, such as in the JET tokamak, the UK center for nuclear fusion research. In this application, SWIR cameras are providing a safety system to check proper confinement of the plasma by monitoring temperature changes within delicate parts of the enclosing toroidal structure.
Figure 2. Inspection of an 18th-century Russian icon using a cooled PSL 320 × 256 SWIR/InGaAs camera. The part of epitrachelion under the hand that blesses is revealed in SWIR image. Photo courtesy of CIK Belgrade.
The surveillance industry is now making use of SWIR cameras, too. Rayleigh scattering of sunlight in the atmosphere – which is responsible for the blue sky and red sunsets, and which causes the blue haze overlying distant scenes – is strongly dependent on the wavelength of the light. Blue is scattered most, and red least. Even further into the red, the longer wavelengths of SWIR are scattered even less by the atmosphere and therefore have an ability to penetrate hazy, misty or smoky conditions, critical in a long-range surveillance system. In addition, SWIR wavelengths are affected less by atmospheric turbulence and heat haze compared with conventional visible-band color cameras (Figure 1).
While traditional thermal cameras are excellent at detecting body heat, they are very poor at providing details for identification. Facial features can’t be made out, nor can writing on signs, for example. In contrast, a SWIR image can appear similar to an image acquired in the visible spectrum. This makes a SWIR camera a powerful surveillance tool.
At night, SWIR imaging comes into its own. Nightglow results in a clear night sky being quite bright in the SWIR spectrum. Reasonable night vision is therefore possible with a SWIR camera. This can be enhanced with near-IR illumination – which, if above 1000 nm, is invisible to all conventional CCD and thermal imagers, and so makes for a simple high-quality covert imaging system. Video delivery via GigE and GenICam compliance is a big asset here, as they greatly simplify camera integration and provide reliable high-frame-rate image delivery in a networked surveillance system.
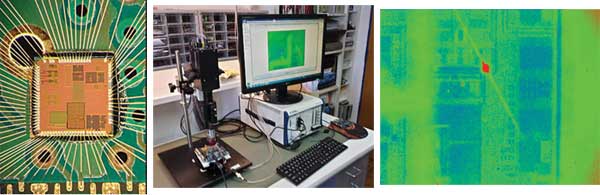
Figure 3. Photoemission captured with a deep-cooled PSL 320 × 256 SWIR/InGaAs camera with a 10-second exposure on a transistor polarized with few-milliampere current, 40-nm technology with seven layers and metal fillings. Photo courtesy of Photonic Science Ltd.
SWIR imaging is also being used to examine the subsurface structure of paintings: The longer wavelengths penetrate the surface layers and can reveal original details that were later painted over. This can help with confirming the artwork’s authenticity, as well as assisting with restoration. In Figure 2, one of the critical requirements for the imaging system is high intrascene dynamic range, so that both the low-intensity parts of the subsurface artwork and surface highlights can be imaged at the same time. The image of an 18th-century Russian icon in Figure 2 shows a good example: Note how the SWIR image reveals the epitrachelion under the praying hand.
Photoemission microscopy is complementing well-known semiconductor failure-analysis techniques such as high-power inspection and microprobing within advanced bipolar transistor production lines. The technique relies on the detection of weak photonic emissions at the 1.1-µm wavelength, which are primarily caused by carrier recombination mechanisms in p-n junctions under forward- or reverse-biased conditions. Standard CCD cameras will capture only the tail of the photoemission peak, resulting in extended exposures, whereas a deep-cooled InGaAs camera sensitive in the SWIR waveband typically will capture an image within 10 seconds. The resulting image is then overlaid with its corresponding die-surface image, so that the emission spot coincides with the precise location of the defect (Figure 3).
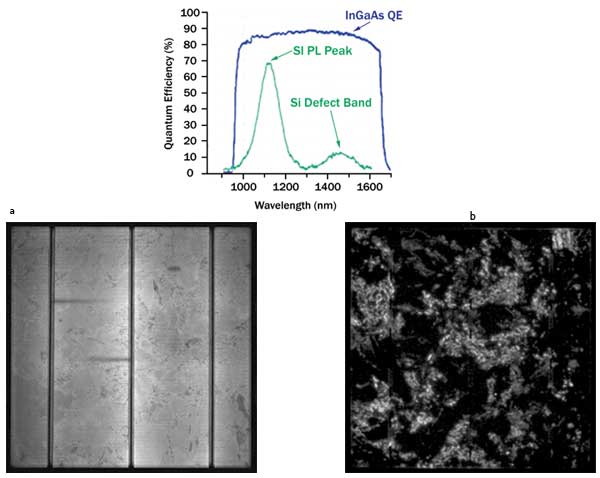
Figure 4. Polycrystalline cells, photoluminescence, imaged at 640 × 512 resolution with a cooled SWIR/InGaAs camera from Photonic Science: (a) 20-ms exposure, primary bandgap emission; (b) 2-ms exposure, secondary bandgap emission. Photo courtesy of GP Solar/GP Inspect.
The same electroluminescence detection technique is applied at the macroscopic level for the detection of defects in solar cells. The forward or reverse bias applied to the solar cell will result in the primary bandgap emission at 1.1 µm as well as secondary bandgap emission beyond 1400 nm. This reveals local defects such as cracks, grain boundaries, broken contacts and shunts, providing a means of identifying and locating abnormal series resistances that will affect the overall maximum photovoltaic current the device can provide.
Photostimulated luminescence, where the solar cell is simply illuminated with light at 800 nm rather than being electrically connected, is also used as a noncontact measurement technique to check the quality of unprocessed wafers at an early stage of production. The image of the resulting photoluminescence at 1100 to 1200 nm in the SWIR waveband reveals the inhomogeneity response due to minority carrier recombination, which is directly related to the future cell-conversion efficiency.
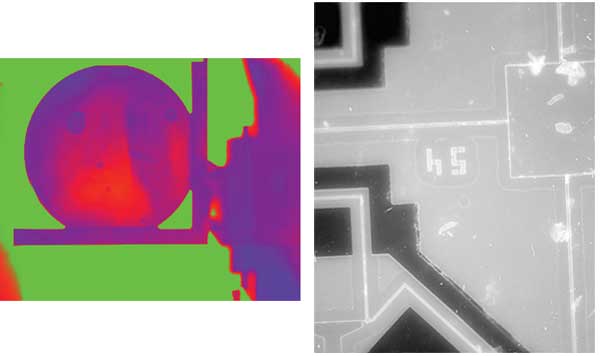
Figure 5. (a) Air-bubble detection within a silicon wafer using a cooled PSL 640 × 512 SWIR/InGaAs camera. (b) MEMS structure imaging.
Unlike traditional CCD cameras, cooled InGaAs cameras have very high quantum efficiency in the photoluminescence waveband and are not sensitive to the visible spectrum. Not only does this mean they have very high sensitivity at 1100 nm, resulting in short exposure times and fast duty cycles, but they also offer the possibility of inspecting from wafer to installed panels under ordinary ambient light conditions (Figure 4).
Another clear advantage of cooled InGaAs cameras is the ability to see through silicon at wavelengths beyond 1200 nm. This helps in identifying structural defects on MEMS or MOEMS devices using suitable backside illumination. The same technique is used to unveil complex 3-D integrated circuit structures at different stages of fabrication, or to identify dislocations within large single-crystal ingots (Figure 5).
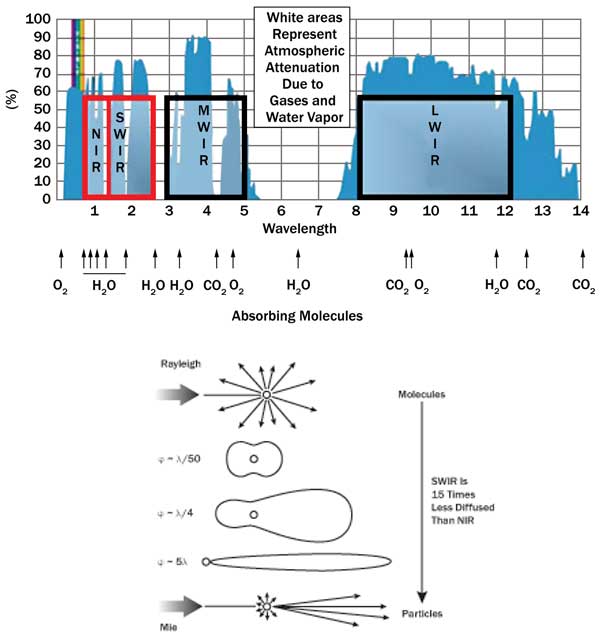
Figure 6. Fluorescence signal emission of different molecules in the IR-spectrum range. Photo courtesy of Photonic Science Ltd.
The benefits of using longer wavelengths than the visible band to “see through” single-crystal structures or large-bandgap materials can also apply to soft tissues and small-animal imaging applications. A typical setup includes a coherent source penetrating through soft tissues, which is generally used to generate a long-wavelength photonic emission from fluorescent markers known as quantum dots or carbon nanotubes. The reduced autofluorescence and scattering process beyond 1300 nm within soft tissues makes it possible to capture faint emissions from the fluorescent markers binding to specific tumors or proteins. Monitoring the fluorescence signal intensity over time provides a means of quantifying protein traffic and drug efficiency. It also can allow the determination of specific gene expression on small animals, with improved signal-to-noise ratio and sensitivity compared with a traditional CCD setup operating at shorter wavelengths (Figure 6).
Meet the author
Scott York is technical director at Photonic Science Ltd. in Robertsbridge, England; email: [email protected].