Bigger aspheric mirrors enable astronomers to see farther into space – but they require special designs.
For astronomers, bigger is better: In general, the scientific value of a telescope scales to the fourth power of its diameter. So, doubling the size boosts scientific worth 16 times – assuming no limiting factors.
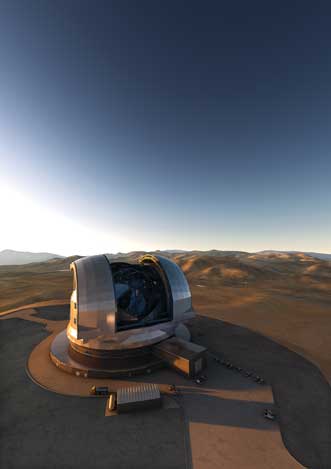
Artist’s impression of the 39-m European Extremely Large Telescope
sitting atop Cerro Armazones in the Chilean Andes. Photo courtesy of
European Southern Observatory
Given that, good times loom. Thanks to photonics innovations and other advances, over the next decade three big telescope projects should come on line. Their primary mirrors range from 25 to nearly 40 m. The two largest current telescopes are about 10 m.
That up-to-quadrupling in size means that scientists can better understand how the universe came to be as it is. Also, extrasolar planets – and their potential for life – can be studied as never before. But that’s only part of the potential inherent in these projects.
“Pretty much every time that we’ve built a bigger, better or just different telescope, we’ve discovered things that we’ve never seen before and nobody ever really imagined or saw coming,” said Jochen Liske, a European Southern Observatory astronomer who is working on the 39-m European Extremely Large Telescope.
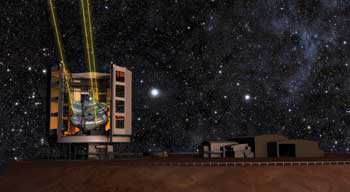
Artist’s rendering of the 25-m Giant Magellan Telescope, showing the seven mirror segments. Lasers are being fired to create artificial guide stars for the adaptive optics. Photo courtesy of Giant Magellan Telescope Organization
The other two projects are the 25-m Giant Magellan Telescope and the aptly named Thirty Meter Telescope. The first two projects are slated for Chile; the third will be in Hawaii; all will be built above 2500 m in places where the air is calm. The wavelengths covered range from 320 to some 28,000 nm, or from the near-UV to the thermal IR.
Bigger mirrors provide two fundamental benefits: First, they collect more photons, enabling examination of very faint
objects. That allows studies of the immediate aftermath of the big bang and the subsequent cosmic evolution, as that in-
formation comes from distant and dim sources. Second, a bigger mirror theoretically means better resolution. This enables extrasolar planets to be directly observed, something difficult today. Importantly, the atmosphere of these alien planets can be analyzed, thereby determining if they contain gases such as oxygen or methane associated with life.
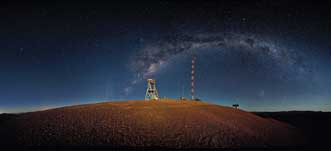
A nighttime panorama of Cerro Armazones in Chile’s Atacama Desert. This is the site for the 39-m European Extremely Large Telescope, in part because the location features calm air and good visibility. Photo courtesy of S. Brunier, European Southern Observatory
These giant mirrors cannot be built out of a single piece of glass because they would sag too much. So they’re built in segments, with the largest being 8.4 m, used in the Giant Magellan Telescope. Although they are constructed out of standard material, they are honeycombed to reduce weight. To cut down on the focal length – and, therefore, the size of the instrument – they’re highly aspheric, departing from a simple spherical shape by many millimeters.
Fabrication of these mirrors is under way at the Steward Observatory Mirror Lab at the University of Arizona in Tucson. Pulling this off required developing unique polishing tools, said Dae Wook Kim, assistant research professor and principal scientist.
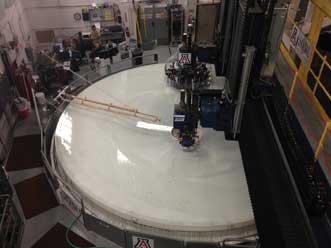
An 8.4-m mirror undergoes polishing to bring it into the right shape at the Steward Observatory Mirror Lab at the University of Arizona. This mirror is the same size as one of the segments of the Giant Magellan Telescope, which will be 25 m overall. Photo courtesy of Dae Wook Kim, UA Mirror Lab
“The tool needs to fit to the surface with extreme accuracy, as the final optical surface needs to be manufactured to better than 20 nm RMS-, or root mean square, shape accuracy,” he said.
Another innovation involves testing how close the surfaces come to the desired shape. Conventional methods don’t work because of the free-form or highly aspheric shape of the surfaces. Therefore, scientists are using a combination of computer-generated holograms, instantaneous phase-shifting interferometers, laser-tracker-aided alignment systems, software-configurable optical test systems and image-morphing software. Together, these methods allow measurement of large surfaces down to the subnanometer, Kim said.
As with other projects, the European Extremely Large Telescope implements a divide-and-conquer strategy, with quasi-
hexagonal sections about 1.4 m in size making up the primary mirror. These must be produced to exacting specifications – and in volume, too, as the mirror will be composed of 798 of them.
“If you look at all the high-performance optics that have been produced in the past for giant telescopes, there were engineering challenges, but you only do a piece or a few pieces,” said Marc Cayrel, project manager for optomechanics. “What is changing now is, because of the number of segments, there is an industrial challenge.”
Fortunately, the project can take advantage of work done to support laser fusion, he added. The need to create high-performance optics to focus petawatt lasers has resulted in the development of techniques to mass-produce mirror segments.
These segments will be precisely and actively positioned by an array of actuators. That array and the other needed technologies have been proved in other telescopes, Cayrel said. Thus, the biggest hurdle may well be ramping up production to create the segments as fast as desired and for the right price.
Prototype segments have already been built, some coming from Paris-based Sagem-REOSC. Roland Geyl, vice president of sales, noted that this confirms the basic production capability. The company also has demonstrated a thin shell for the adaptive optics used on the fourth mirror, or M4, of the telescope. It measures 2.5 m across and is only 2 mm thick, making it the world’s largest, Geyl said.
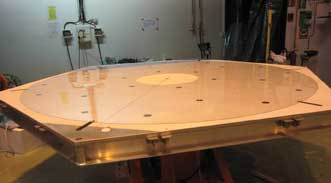
Flexible thin shells that measure meters across and millimeters thick form the basis for an adaptive optics implementation that will correct for atmospheric turbulence in next-generation large telescopes. Adaptive optics allow ground-based instruments to image at their diffraction limit. Photo courtesy of SAFRAN-Sagem-REOSC
It isn’t only the size of the new telescopes that is important. The adaptive optics also are critical. For instance,
some 6000 actuators will sit behind the thin-shell M4 mirror in the European Extremely Large Telescope. These will push against the shell, deforming it many times a second in a precise fashion. Those shape changes will cancel out atmospheric turbulence and other distortions of the incoming photon wavefront. The result will be resolution that approaches the theoretical diffraction limit.
Thus, the stars as seen through the telescope won’t twinkle. They will be about as sharp and with as high a contrast as possible. This ability to maximize contrast and resolution makes it possible to study extrasolar planets.
Telling the actuators how to move can be tricky because what is needed is a relatively large number of photons traveling the same path as those from the object of interest. That need for a guide star can be hard to meet for great patches of the sky, but solutions have been devised, said Larry Stepp, telescope department head for the Thirty Meter Telescope in Hawaii.
“There is a sodium layer in the atmosphere that is about 90 km above the ground. It has been discovered that, if
you shine a laser with the right frequency to excite the sodium ions, you can make a glowing spot up at that altitude. That can then be used to put a guide star wherever you want,” he said.
That technique has been extended to multiconjugate adaptive optics, systems that use multiple deformable mirrors to correct turbulence at different heights. Such an approach improves and broadens the correction to cover a larger field of view.
At present, adaptive optics only routinely correct to the diffraction limit down to near-IR wavelengths, or a micron. Some success has been achieved down into the red, or about 600 nm. In general, going shorter in wavelength demands more actuators, more powerful lasers and faster systems. Further technology advances are anticipated to extend adaptive optics capabilities over the telescopes’ lifetimes, which should run into the decades.
As for instrumentation, those that use uncorrected light tend to scale with the size of the telescope. Thus, they can become quite large, expensive and challenging to build, said Patrick McCarthy, Giant Magellan Telescope project director.
Those that can make use of wavelengths improved by adaptive optics tend to be more manageable in size, simply because a bigger telescope will produce a sharper image. However, a bigger telescope can present other challenges because it can capture photons over a bigger swath of the sky. That may mean the instrument will require more pixels, something difficult to do if the number is too large.
One solution would be to break the instrument load down into smaller pieces. An example might be a multiplexing spectrograph, which examines the light from hundreds of objects at one time. This is accomplished via optical fibers directing light to many different detectors simultaneously.
Bursts of telescope building tend to happen when clever ways are found to overcome previous limitations, McCarthy noted. Recent advances have made it possible to construct telescopes at the 30-m scale or even larger, but there may also be another reason for the building boom.
“There’s a big push to take advantage of this technological window,” he said. “And I think what we’re seeing is the worldwide community of countries that have the technical and scientific wherewithal to do this has gotten much larger.”
Why Chile and Hawaii?
Although modern technology can boost a telescope’s performance, putting these multibillion-dollar machines in the best possible location helps, too. Getting above the atmosphere is an advantage. Another plus is a steady laminar air flow. Thus, the best places on Earth to do astronomy are coastal mountain ranges and isolated volcanic islands.
“When you fold in light pollution and other issues, you really wind up with the coastal range of Chile or Hawaii as being the two best astronomical sites in the world,” said Patrick McCarthy, Giant Magellan Telescope project director.
For the project McCarthy leads, the decision to build in Chile was influenced by this and the fact that the center of the Milky Way passes overhead.