Rush Laselle, !%Adept Technology Inc.%!
The global photovoltaic
(PV) manufacturing community is on the cusp of a resurgence in investment, development
and innovation, a revolution that largely will be driven by technology. It is vital
to find the most effective – and most cost-effective – tools and processes
to increase productivity and decrease costs within a set capital plan. Robotic automation
is a significant part of solar cell manufacturing, but it is important to consider
which robot types and kinematics are best for each unique process, looking at the
solar manufacturing areas where there are the greatest return opportunities for
robotic automation as well as investigating which robot type is best for a particular
solar application task and where vision fits.
In this article, I will provide a primer targeting these issues
and discuss how the solar industry can best maximize factory throughput, reduce
costs and improve efficiencies with robotic automation.
Flexibility through vision
Vision has become a highly adopted tool to improve the productivity
of robotic automation in all industries and all facets of placement. Vision systems
offer tremendous flexibility for applications that don’t require fixtures
or trays for part location. Vision guidance enables the system to take a picture,
compute a part’s location and orientation, and guide the robot to the part
using a computed robot-to-camera transformation obtained through an automated calibration
process. Tremendous flexibility and cost savings are realized because parts don’t
have to be fixtured.
Vision systems allow parts to be randomly presented to the robot
without orientation or alignment, or without being placed in a tray, which also
reduces cost. These systems frequently incorporate line tracking, enabling the robot
to pick these parts from a moving belt, further optimizing the process.
Robot-integrated vision allows inspection to be incorporated right
into the handling process, placing it in parallel with handling, further reducing
the overall cycle time and increasing throughput. Various part geometries require
only vision retraining or the selection of a recipe instead of manual changes in
fixtures and tooling. This increases the overall lifetime profit of the equipment
by virtue of its optimization and improved throughput. Most robot manufacturers
offer packages with multiple cameras and tracking solutions for integration into
a single cell. This offers tremendous power and flexibility for solar manufacturing.
The right kinematic solution
How do you select the right robot for the task? First, you must
consider the payload requirement for the robot. People often consider only the products
being handled. But it is also important to consider the tooling solution or end-of-arm
tooling (EOAT).
Evaluating the motion requirements also is critical. Not only
the simple motion of picking and placing but also what interferences exist between
the robot and its linkages as well as other items that may be in dynamic motion
within the cell must be considered.
How repeatable must the robot be? Robot manufacturers speak in
terms of repeatability, while engineers and designers look at accuracy. A robot’s
repeatability outlines the machine’s ability, once programmed, to return to
the taught position. Accuracy refers to the ability to input a given location digitally
and to have the robot move to that point in space “accurately.” This
encompasses offsets and other digitally input motion parameters, often varying within
a given mechanical unit’s work envelope. Thus, you must make sure you carefully
evaluate these factors to gain a good understanding of the requirements of a process
plus the capabilities of a given robotic solution.
Do your processes require special environmental considerations?
Do you need a robot designed to eliminate the generation of particulates that might
degrade the product? Or must the robot be protected from process-specific elements
such as those in slurry ingot processing?
Robot kinematics
The four major categories of robot kinematics are Cartesian, SCARA
(selective compliant assembly robot arm), articulated and delta/parallel.
The Cartesian kinematic solution is simple and highly configurable
(Figure 1). The platform includes everything from a single degree of freedom or
unidirectional travel to numerous axes of motion. Adjusting strokes or lengths and
configuration is relatively easy with Cartesian kinematic solutions as compared
with the other types. Multiple drivetrains exist, optimized to provide high throughput
or precise motion as characterized by whether the drive might be a ball screw or
a belt-driven mechanism.
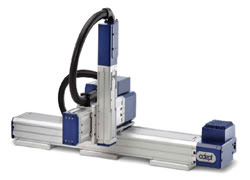
Figure 1. Cartesian solutions are typically called upon to serve
applications where the substrate remains in the same plane. Images courtesy of Adept
Technology.
Platforms exist to accommodate small-part assembly up to extremely
large part transfer such as overhead cranes that might be observed in a manufacturing
facility. In the photovoltaics industry, Cartesian solutions can be applied to both
small and large work spaces. They typically serve applications where the substrate
remains in the same plane and does not have to be flipped or have its configuration
changed, other than a rotation in the same plane as the table or conveyor (X-Y plane).
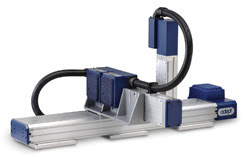
Figure 2. Within solar manufacturing processes, SCARA robots are best suited for high-speed
and high-repeatability handling of cells in smaller work spaces.
The next robot is the SCARA, which offers a cylindrical work envelope
and typically provides higher speeds for picking, placing and handling processes
than do Cartesian or articulated robotic solutions (Figure 3). It also delivers
greater repeatability by offering positional capabilities superior in many cases
to articulated arms. This class of robot usually is used for lighter payloads in
the sub-10-kg category for assembly, packaging and materials handling. For solar
manufacturing processes, it is best suited for handling of cells in smaller work
spaces with high speed and repeatability.
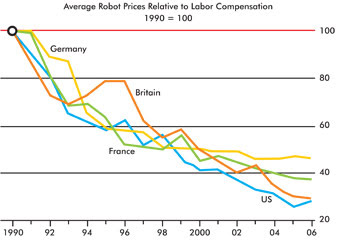
Figure 3. With more automation, the solar
industry can potentially realize a 75 percent savings in direct labor costs alone.
Courtesy of International Federation of Robotics.
Articulated robots, the third group, have a spherical work envelope,
and their arms offer the greatest level of flexibility because of their articulation
and higher degrees of freedom (Figure 4). Because they make up the largest segment
of robots available, you can find a wide range of solutions, from tabletops to large
1000-kg-plus solutions. Articulated robots are applied to a variety of solar applications,
including the handling of heavy silicon ingots or glass, or the handling of subassemblies
or assemblies where the products are introduced to the cell in a configuration different
from the way in which they are presented to the system.
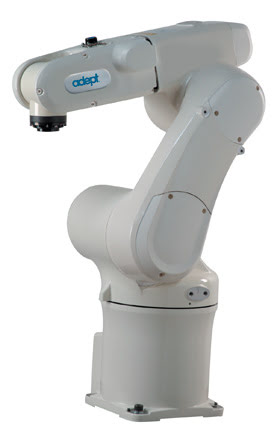
Figure 4. Articulated robots are frequently
applied to process intensive applications where they can use their full articulation
and dexterity for solar applications such as handling silicon ingots, glass, subassemblies
and assemblies.
Delta/parallel robots make up the fourth category (Figure 5).
This kinematic solution provides a cylindrical work envelope and is most frequently
applied to applications where the product again remains in the same plane from pick
to place. The design uses a parallelogram and produces three purely translational
degrees of freedom, so work must be done within the same plane. Base-mounted motors
and low mass links allow for exceptionally fast acceleration and, therefore, greater
throughput over the other groups. An overhead-mounted solution, it maximizes access
but also minimizes footprint. These units are designed for high-speed handling of
lightweight products and offer lower maintenance because of the elimination of cable
harnesses and cyclical loading.
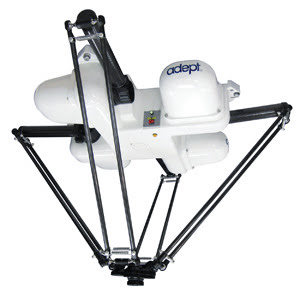
Figure 5. Parallel robots offer high-speed transfer of solar cells through manufacturer
lines and a multitude of processes.
Parallel robots are deployed into many solar cell processing steps
because they offer high-speed transfer of solar cells through manufacturer lines
and a multitude of processes, including diffusion of process equipment, wet benches
and PECVD (plasma-enhanced chemical vapor deposition) antireflection coating machines.
The Quattro parallel-linked product from Adept Technology Inc. recently achieved
300 cycles per minute, illustrating the capabilities for this class of machine to
handle products at high rates.
Deployment within the solar process
Typical PV process steps can be seen in Figure 6. The steps are
broken into four basic groups where high concentrations of robots are deployed.
The ingot processing step predominantly uses Cartesian gantries and large articulated
arms because of the requirement for heavier payloads and large work-space optimization.
Wafer manufacturing uses a variety of arm types, depending upon volume and process
requirements. Cell processing tends to use gantries, SCARAs and parallel linked
robots. Reach and repeatability considerations usually are the deciding factors.
Module building uses a variety of arms – often articulated and Cartesian arms
for reach and flexibility, although some specific tasks use the services of SCARAs
and parallel robots.
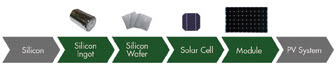
Figure 6. High concentrations of robots
are being deployed into the four basic photovoltaic process steps.
Antireflection coating process
Comparing the four robot categories, we consider their usefulness
for an antireflection coating load/unload process. A Cartesian robot is optimized
from a reach standpoint. However, the majority of solutions here would prove too
slow and would require in excess of a single-head EOAT tooling. Because this complication
would drive the need for prealignment and could result in further complications
in preconditioning the product, a Cartesian solution could be considered less flexible.
Cartesian robots
• Optimized for reach
• Too slow for loading/unloading using a single-head EOAT
• Because multihead EOAT often is used, cells require prealignment.
• Less flexible when reconfiguring for different size wafers is required
SCARA robots enable increased speeds and are more flexible than
Cartesians. However, in a traditional tabletop version, the work space is limited,
so SCARA may not be optimal in reaching all points on the load and unload areas.
SCARA robots
• Faster and more flexible than Cartesian robots when used with vision guidance
• Table-mounted versions could limit work space, and multiple robots may be required to cover pallet/matrix.
Articulated robots would be pedestal-mounted and could prove too
slow in increasing the complexity of the installation.
Articulated robots
• Too slow for loading/unloading with single-head EOAT
• Spherical work envelope isn’t ideal for covering pallet/matrix.
The optimal choice might be a delta or parallel-style robot, for
a number of reasons. The overhead mount is ideal for reducing the footprint of the
automation cell. It can reach all places on the PECVD pallets. And when the benefits
of the delta are combined with vision, it provides an exceedingly flexible solution
that will meet the throughput requirements. Note: Vision is an enabler not only
for parallel linked robots but for all categories of robots.
Delta/parallel robots
• Overhead mount design is ideal for loading/unloading equipment.
• Larger delta robots can cover the width of most PECVD pallets.
• When used with vision guidance, they enable extremely good positioning.
• Excellent flexibility and quickly reconfigurable
• Robot design is optimal for handling cells (lightweight) at high speeds.
Conclusion
The common goal for solar manufacturers is to drive down the cost
per watt. As the solar industry strives to achieve grid parity, manufacturers must
be knowledgeable about modern robotics as well as about automation and vision technologies
– and the value they contribute to helping reduce the cost of solar cells.
History has shown that automation plays a significant role in
reducing manufacturing costs in many industries, and when the costs associated with
higher quality and yields are considered, its benefits offer an even more appealing
value proposition. Although robotics and automation may be viewed by some industries
as mature technologies, industry leaders are continuing to develop innovative products
and new technologies that are ideal for solar manufacturing processes.
It would be prudent for solar manufacturers to look outside of
their industry for the best practices in high-volume manufacturing with automation,
robotics and vision to achieve cost-reduction goals.
Meet the author
Rush LaSelle is director of worldwide sales and marketing at Adept
Technology Inc. in Pleasanton, Calif.; e-mail: [email protected].