Rapid detection of light calls for a sensitive detector and electronics that do not introduce additional signal noise. In fact, for very rapid detection, detector shot noise does not determine the signal-to-noise ratio. Instead, the amplifier has the most impact, which is why many designers have selected a photomultiplier tube for the high-speed or low-light-level detection of photons.
Ken Kaufmann, Hamamatsu Corporation
The applications where rapid detection of light is a must are many. They include a wide variety: semiconductor inspection, gene sequencing, oil well logging and high-energy physics. The reasons for high-speed detection are varied, but often the motivation is either high sample throughput or short signal life. Detection at high speed often means that the total number of detected photons is very small. Basic requirements include a sensitive detector and electronics that do not add to signal noise. A photomultiplier tube (PMT) that can detect single photons and lessen the signal-to-noise problem fills the bill. (A thorough discussion of the signal-to-noise ratio of optical detection systems can be found in “Detectors: Guideposts on the Road to Selection”).
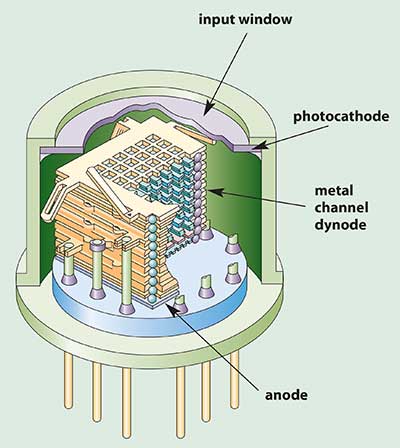
Figure 1. The basic photomultiplier is both simple and complex.
To understand how a PMT detects single photons and how it amplifies an optical signal with virtually no additional noise, we need to understand how one is constructed and how it functions.
In a PMT (Figure 1), light enters through the input window and strikes a photocathode, which converts the photon to a photoelectron.
The active area of the photocathode can be as small as a few millimeters in diameter or as large as a sphere half a meter in diameter. Such a large variation in size is important in optimizing the sensitivity, cost and dark current — all of which are proportional to the size of the active area.
Material choices
Photocathodes can be made from either alkali metals or semiconductors. The wavelength range over which one responds to light can be adjusted by changing the cathode’s chemical composition. Figure 2 illustrates typical response curves for PMTs with reflective photocathodes for optical signals from 140 to 1200 nm.

Figure 2. The spectral response of a photomultiplier covers a wide wavelength range.
The photocathode can be constructed to cover a limited portion of the optical spectrum. A solar blind photocathode can be manufactured to provide excellent sensitivity to ultraviolet light, but no response to daylight. Such cathodes are useful for flame detection, especially for flames such as those created by burning hydrogen in which no visible light is emitted.
A second example of photocathode selectivity is the sensitivity of bialkali photocathodes to the near-ultraviolet and visible regions only (300 to 700 nm). PMTs with this type of photocathode are particularly suited for coupling to NaI(Tl) scintillators for scintillation counting because the cathode's wavelength sensitivity matches the emission of the scintillator. The bialkali's sensitivity has been further enhanced in "super bialkali" and "ultra bialkali" photocathodes, which are manufactured to have quantum efficiencies as high as 35 percent and 43 percent, respectively, at peak wavelengths.
Another example of photocathode engineering is a new semiconductor device that operates in the near-infrared with high sensitivity out to 1700 nm. Applications that require sensitivity in the infrared only are being developed. One application that is of great interest is the detection of the very weak emission from singlet oxygen. Singlet oxygen is a highly reactive compound believed to play an important role in the destruction of brain cells immediately after a stroke, and in the photochemical damage of plastics and other organic compounds that are exposed to sunlight or ultraviolet radiation.
All photocathodes produce an electron by the photoelectric effect. This photoelectron is very difficult to detect and needs to be amplified by factors of a hundred thousand or a million before it can be processed. This can be done inside the PMT without relying on external amplifiers.
Amplification is carried out using the dynode chain, a series of eight to 12 metal plates. A potential of around 100 V is applied between the photocathode and the first dynode. The photoelectron is then accelerated into the dynode. Upon striking the dynode, five to six secondary electrons are produced. These electrons are accelerated into the second dynode by a potential difference of about 100 V producing between 25 and 36 electrons.
As the electrons continue cascading from one dynode to another (with a potential difference of around 100 V), an electron pulse of between 100,000 and 1,500,000 is produced. This large gain in signal is obtained with almost no additional noise because the noise figure is 1.2 for a typical PMT. By comparison, the noise figure would be 1 for an ideal amplifier. As an additional benefit, this gain is obtained without sacrificing bandwidth. PMTs can have bandwidths up to 1500 MHz. A bandwidth of 100 MHz is very common.
Shape is preserved
After exiting the last dynode, the electron pulse is collected on the anode. Careful design of the shape of the dynodes and the large electric fields between them results in an output pulse on the anode that can be as short as 1 to 2 ns, thereby preserving the temporal shape of the initial optical signal. The very high internal gain generates a current pulse resulting from the single photon that can be as large as 100 µA. Into a 50-Ω load this results in a signal of 5 mV. Often, this can be detected without further amplification.
For most measurements, the low noise figure and high bandwidth guarantee that the detector will not distort the signal. Thus, it is possible to detect both very brief and very weak phenomena.
When the rate at which photons strike the photocathode is below 100 MHz, it is possible to count each anode pulse, or, in other words, to process the signals digitally. As the rate increases above this level, it is necessary to use analog methods such as integrators or sample-and-hold circuits to process the electrical signal.
Even with analog signal processing, the dynamic range of the photomultiplier can be as large as five orders of magnitude, thereby allowing the circuit designer a great deal of latitude in the size of the signal that will be detected. While photon counting is the preferred method of signal acquisition, analog signal processing can be implemented once the photon flux is above 10,000 per second.
Size is important
While the PMT may have been in use for more than half a century, the technology itself is constantly evolving. PMTs now can be made no larger than the TO8 that is used for photodiodes. These small, very sensitive detectors can be coupled to a fiber, or a plastic lens can be attached to their input window to increase the effective active area by 50 percent.
A small detector is not very useful if the power supply for the photocathodes and dynodes is large. Active power supplies using the Cockroft-Walton design can be made to fit into a case smaller than a TV remote control. Because it generates high voltage internally, the Cockroft-Walton design has the added benefit of not requiring high-voltage input. Thus, it is possible to produce a high-sensitivity detection system that is compact and can be powered with a 9-V battery.
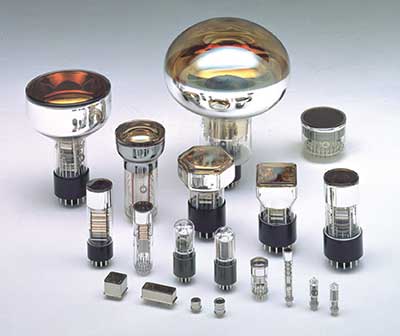
Figure 3. Photomultipliers come in a variety of shapes and sizes.
One application for such a compact detector is as a portable hygiene monitor. Here, a food preparation area is wiped with a swab treated with a chemical to break open cells, such as bacteria, which release their adenosine triphosphate (ATP). The swab is then placed in a hygiene monitor where the ATP reacts with luciferin to generate chemiluminescence. While the emitted luminescence is weak, it is easily detected by a PMT.
PMTs are also extremely reliable and robust. In many applications, the MTF is in excess of 10,000 hours. Modern oil drilling methods require the drill to maneuver so that it can be directed toward the oil reservoir. Such a drill requires a detection system that can monitor the composition of the rock. Gamma-ray spectroscopy is used for measurement while drilling to detect the presence of oil-bearing rock. PMTs coupled to scintillators are used to detect gamma rays scattered by the surrounding rock. The size of the scattered signal determines rock density and thereby detects an oil deposit. During the drilling process, the PMT is subject to shock as high as 1000 g, vibration of 50 g, and temperatures of 180 °C.
Hybrid detectors
Besides PMTs, another type of photomultiplier — the hybrid photodetector (HPD) — can detect low levels of light. The HPD, a device which combines elements from PMTs and photodiodes, is a vacuum tube with a photocathode and a silicon avalanche diode. It functions similarly to a PMT but with a different mechanism of amplification.
After light strikes the photocathode and is converted to a photoelectron, the photoelectron is accelerated towards the avalanche diode to be amplified in two steps. Upon collision with the diode, the photoelectron produces many electron-hole pairs (typically greater than 1000) within the silicon, resulting in "electron-bombardment" gain. These electrons then drift to the avalanche region, where they create more electron-hole pairs in a series of chain reactions (avalanche gain). The product of the electron bombardment and avalanche gains is the total gain, typically greater than 105.
Due to the HPD's amplification mechanism, this device has a better pulse height resolution than a PMT, allowing it to detect single and multiple (up to 4 or 5) photons. In addition, this device offers resistance to magnetic fields, high-speed detection, and low afterpulses. The HPD is suitable for a variety of applications including light detection and ranging, time-correlated single photon counting, high-energy physics, and fluorescence correlation spectroscopy.
The photomultiplier is a versatile and sensitive light detector that can be used in many applications, especially where light levels are low or speed is important.