Performance of a photodetector with respect to the four basic attributes of light, in addition to cost, can be used to make an appropriate choice.
Earl Hergert and Slawomir Piatek, Hamamatsu Corporation
Light is a versatile tool for investigating physical and chemical processes in nature. Any specific system being analyzed may, through the light it emits or reflects, communicate information about itself. Electrical signal is an effective medium for processing, transporting, and storing information. The photodetector’s role is to convert information propagating in the form of light into an electrical signal with a minimal loss of information quality.
Information carried by light may be encoded in any combination of light’s four basic attributes: spectral composition (W), intensity (I), and temporal (T) and spatial (S) characteristics (Figure 1). Thus the choice of the appropriate photodetector hinges on its performance with respect to these four aspects and, in addition, cost ($) — altogether known as WITS$.
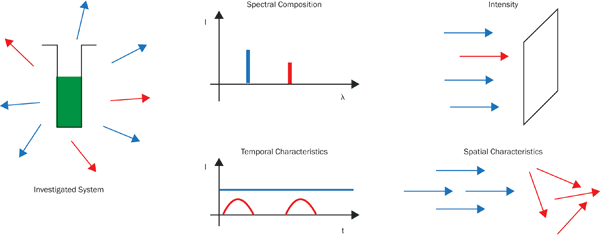
Figure 1. Light from the system under investigation may contain information about the system in the form of spectral composition, intensity, temporal characteristics (e.g., constant intensity, pulses, exponential decay, etc.), and spatial characteristics (e.g., collimated light, diffuse light, etc.).
The signal-to-noise ratio (S/N) at the output of a photodetector is the measure of the detector’s performance and the figure of merit when choosing from an available pool of detectors. This selection guide is for a set of four point detectors: photomultiplier tube (PMT), photodiode (PD), avalanche photodiode (APD), and silicon photomultiplier (SiPM).
A PMT is a vacuum tube with a light-sensitive photocathode, a sequence of dynodes providing an internal electrical gain and a signal-collecting anode. A PMT is characterized by low internal noise, a gain (μ) on the order of 105 to 106 and an ability to detect single photons. A PD is a light-sensitive, solid-state device with a p-n junction and no internal gain (μ = 1). In a typical operation, a PD is reverse-biased to several volts. An APD is a type of a PD that has an internal gain. In a typical operation, an APD is reverse-biased to a voltage that is close to but less than its breakdown voltage. A gain of up to about 100 is possible. If the reverse voltage is less than the breakdown voltage, an avalanche triggered by a single charge carrier quenches by itself; otherwise, the avalanche must be externally quenched. The latter mode of operation is known as the Geiger mode. An SiPM is a rectangular array of square cells, or pixels, where each cell is a series combination of an APD and a quenching resistor. All of the cells are in a parallel combination. In a typical operation, an SiPM is reverse-biased so that the APDs operate in Geiger mode, giving the device a gain that is comparable to that of a PMT. One may think of an SiPM as a solid-state analog version of a PMT, albeit with higher internal noise. Table 1 compares the four photodetectors.
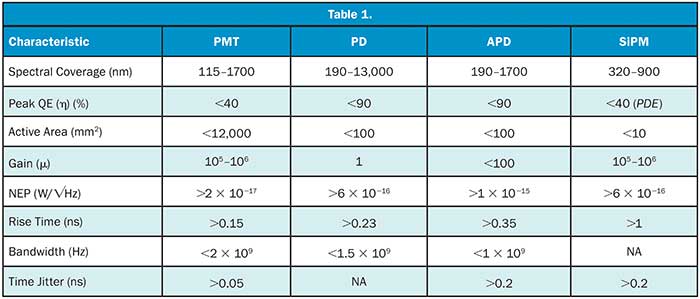
Selection based on wavelength
The first step in the selection process is matching the spectrum of the observed light with the spectral sensitivity of a potential photodetector. The pertinent characteristic to be inspected is the detector’s quantum efficiency as a function of wavelength η(λ) or, alternatively, responsivity σ(λ). Quantum efficiency is a ratio of the number of photogenerated charge carriers per unit time contributing to the output signal to the number of incident photons on the light-sensitive section of the photodetector per unit time. Responsivity is a quotient of the electric current, I (in amperes), resulting from the photoconversion and the incident optical power, P (in watts). For a given wavelength, the two quantities are related by η = 1240σ(A/W)/λ(nm). A device manufacturer provides at least one of these two characteristics in the form of a plot as a function of wavelength. Based on the W criteria only, the responsivity of the optimal photodetector would be higher than that of a competing photodetector at the wavelength of observed light. Ideally, this responsivity would also be close to, if not equal to, the peak responsivity of the optimal detector. If a photodetector has zero responsivity at the wavelength of interest, the detector must not be selected regardless of its other characteristics.
Photon detection efficiency (PDE) is an attribute of an SiPM that expresses a fraction of the incoming photons contributing to the output signal. It is a product of η(λ), fill factor (i.e., the ratio of the light-sensitive area to the total area) and the probability of avalanche. A manufacturer provides plots of PDE as a function of wavelength and overvoltage (i.e., bias voltage minus breakdown voltage). If PDE is zero at the wavelength of interest, an SiPM is not an appropriate selection.
The first row in Table 1 gives the spectral coverage for the four families of photodetectors. None has photosensitivity for λ < 115 nm. However, using scintillation techniques could make detection of such radiation possible. PDs have the widest spectral coverage: their photosensitivity extends into far-infrared. Operating in this spectral region almost always requires cooling — in some cases, to a temperature as low as 4 K.
The second row in Table 1 lists the peak quantum efficiency. The physical structure of the device and the type of light-sensitive material used in making the device control the wavelength of maximum quantum efficiency. The values show that solid-state devices have higher peak quantum efficiency when compared with PMTs.
Selection based on intensity
The second step in the selection process is estimating the S/N at the output of the photodetector for the given intensity of incident light, which is an important factor the potential user needs to know — at least approximately. The desired outcome is the highest possible S/N.
There are two definitions of radiant intensity used in literature, which are not equivalent. In the first definition, it is the radiant power per unit solid angle and in the second, it is the radiant power passing a unit area. The latter is more appropriately known as irradiance, or I (in W•m−2). Suppose that a monochromatic light with constant irradiance illuminates a photodetector with active area, A. The rate (nγ) with which the photons arrive at the surface is:
Within this equation, Eγ = hc/λ is the energy of a single photon; P = IA is the power of the incident light; h is Planck’s constant, and c is the speed of light. The resulting rate of charge carrier photogeneration in the detector is given by:
The output signal from a photodetector can be viewed as a chronological sequence of current pulses, each originating from a single photogenerated charge carrier. Together, equations 1 and 2 imply that ne depends linearly on the product of I and A. Maximizing S/N may consist of maximizing the signal, S, and/or minimizing the noise, N. For a given irradiance, increasing the signal may be possible by increasing incident light power with light-collection optics or by selecting a photodetector with a larger active area. Decreasing the noise may be possible by selecting a detector with lower inherent noise and/or by limiting detection bandwidth. Manufacturers provide guides, sometimes both in print and as an online resource, on how to estimate the minimum detectable power — an input optical power yielding a S/N = 1 at the output of the detector. A spec sheet for a photodetector also is likely to list noise equivalent power (NEP) in units of W/√Hz, which is the incident optical power that generates a photocurrent equal to the root mean square (rms) noise current for 1-Hz bandwidth. NEP is useful when comparing photodetectors and, all else being equal, a photodetector with a lower NEP yields a higher S/N output.

It is important to note several points: 1) A complete estimate of S/N should include the detection circuit’s contribution to the noise (e.g., noise caused by a resistor or by a transimpedance amplifier). This contribution becomes less significant for a photodetector with internal gain, and this fact alone is the reason for a gain in a photodetector. 2) The minimum detectable power is a function of detection bandwidth. Higher bandwidth increases noise and, therefore, increases the minimum detectable power. Alternatively, higher bandwidth lowers S/N for a given power of input light. 3) Large bandwidth is desirable if high fidelity is required — the output electrical signal accurately reproduces the input light signal. 4) The minimum detectable power for a given bandwidth is always larger than NEP for the same bandwidth. 5) Terminal capacitance of the photodetector affects the detection bandwidth — the higher the capacitance, the smaller the bandwidth. 6) For solid-state photodetectors (but not for PMTs), a larger active area causes a larger terminal capacitance, which decreases the detection bandwidth. Consequently, there is a tradeoff between sensitivity and bandwidth, or sensitivity and signal fidelity.
In summation, the incident light power must be greater than the minimum detectable power for the photodetector to be a viable selection.
Figure 2 depicts the suitability of selecting a photodetector depending on the input light level. In it, the scaling for the top axis corresponds to the photon irradiance (number of photons•mm−2•s−1). The axis below gives the corresponding irradiance (in W•mm−2), assuming monochromatic light with λ = 550 nm. The bottom axis gives a distance, R, in meters, from a 1-W point source producing the above irradiances. Suppose that each photodetector has an active area equal to or greater than 1 mm2, and that it is spot illuminated with an effective beam size of 1 mm2. In this case, the rate of the photon arrival nγ is equal to 1 mm2 times the photon irradiance. If nγ is less than about 108 s−1, the current pulses are distinguishable in time, and individually detecting them is known as “photon counting.” The horizontal red line in the figure depicts this regime. Blue arrows indicate the range of irradiances that each respective photodetector can detect.
Figure 2. Photodetector suitability as a function of irradiance.
A PMT is the best choice — perhaps the only choice — for situations where the incident light level is extremely low. As the light level increases, SiPM, APD, and PD become viable choices. Where the detectors overlap, other light and detector characteristics should be considered in making the selection. For high light levels, a PD and an APD are the possible choices. However, in a vast number of cases, a PD will be the better choice when ease of use and device cost are taken into consideration.
Selection based on temporal characteristics of incident light
The intensity of the incident light can be a function of time. It is a “DC light” if the intensity is constant, “AC light” if the intensity varies with time, and “pulsed light” if it arrives as discrete packets of photons. DC light poses no additional restrictions on the photodetector, whereas the other two do impose restrictions. For AC light and pulsed light, capacitances (e.g., junction, parasitic, or terminal) matter — their values affect the output signal rise time, time jitter, and detection bandwidth. Table 1 lists these parameters: the rise time and bandwidth are for a 50-Ω resistive load (current-to-voltage conversion), whereas time jitter is for a single-photon illumination. Increasing the load resistance increases the output voltage but reduces the bandwidth. Because the quantity of noise in the output increases with bandwidth, a well-designed system has just enough bandwidth for the purpose of the observation.
Dynamic range and a related concept — linearity of a photodetector — should also be examined in pulsed and AC detection. Is there enough dynamic range to detect the full variation of the incident intensity? Are the input and output signals linearly related? If not, what is the deviation, and is it acceptable? Several factors can influence the dynamic range of a photodetector, such as an intrinsic noise floor, biasing level, output load and, in the case of an SiPM, number of cells. Manufacturers most commonly provide information on dynamic range and linearity in the form of graphs, examples of which may be linearity versus the power of incident light or linearity versus the load resistance.
Selection based on spatial characteristic of incident light
If the level of incoming light is low, but the light is nearly collimated, employing focusing optics can increase the incident light power on the detector and thus improve the S/N. However, if the incoming light is diffuse, focusing optics will not increase the incident power, as diffuse light cannot be focused. The only other option is to use a detector with a larger active area. The tradeoff, though, is a higher dark current in the photodetector, which increases noise and, therefore, NEP. In the respective cases of a PD, an APD, and an SiPM (but not a PMT), a larger active area reduces the detection bandwidth due to a larger junction capacitance.
Selection based on price
If the WITS$-based selection process does not produce a unique and outstanding choice (which is unlikely yet possible), then price may be the deciding factor. Prices can vary greatly among the different models of a photodetector in a given family, but when the typical representatives of the families are compared, the highest-to-lowest pricing is, respectively, PMT, SiPM, APD, and PD. This is a price for a stand-alone photodetector. If the potential user needs to design a detection setup from the ground up, the cost of auxiliary equipment (e.g., power supplies and amplifiers) should also be considered. All of the detectors described in this article are also offered as part of a module. Inclusive of the photodetector, a module may or may not contain, among other components, a power supply, amplifier, temperature and/or voltage control system, or an A-to-D converter.
In most cases, the selection process ends here. There are, however, applications and situations where additional criteria need to be considered. Examples include such criteria as ruggedness, immunity to an external magnetic field, ease of use, physical size, and ease of customization. If the device may be customized, how much might it cost to do so? How long might the lead time be?
The selection process can be taxing, confusing, and potentially costly. Establishing a partnership with the device manufacturer can make the process much quicker and result in optimal sensor selection.