As femtosecond lasers grow more versatile, more stable, and easier to use, their end markets are expanding and multiplying.
MARIE FREEBODY, CONTRIBUTING EDITOR
Ultrashort pulse lasers, such as femtosecond lasers, are becoming increasingly easy-to-use plug and play devices suitable for a wide range of industrial and biomedical applications. Fifteen years ago, these lasers were large mainframe affairs requiring daily cleaning of the optics, regular maintenance of water for cooling, and constant optimization of laser parameters.
Today, solid-state and fiber-based
platforms using well-established photonic-crystal fiber amplification technology and chirped pulse amplification architectures have produced femtosecond lasers that are compact, highly reliable, and less expensive.

A femtosecond fiber laser laboratory setup for the generation of a broadband frequency comb in the visible spectral range. Courtesy of TOPTICA Photonics.
Within today’s ultrashort sources, the laser cavity is airtight, with even the larger disk or slab amplification cavities enclosed for more effective isolation from the environment. This means that modern ultrafast lasers no longer require manual alignment in the field, and they are less affected by changes in temperature or humidity.
“A few decades ago, it used to be that if you sneezed too hard next to an ultrafast laser, it could become misaligned or lose mode-locking,” said Heather George, a product manager at TRUMPF. “The advent of passive mode-locking seed lasers rather than active mode-locking seed lasers made industrial ultrafast lasers possible.”
Turnkey femtosecond laser sources now offer various price points as well as flexible pulse durations, pulse energies, average powers, and beam parameters.

The ultrashort pulses of femtosecond lasers can ablate or mark materials with minimal heat damage, enabling their use as effective tools for processing heat-sensitive materials. Courtesy of TRUMPF.
Many of these systems further incorporate functionalities such as automated pre-compensation of unwanted group velocity dispersion or integrated acousto-optic modulators that allow control over average power, energy per pulse, and repetition rate.
“Apart from small and slow performance degradation related to natural component aging, a turnkey laser should not require any periodic adjustment or
re-optimization on part of the user, and
it should have remote diagnostics capabilities and offer remote service intervention to maximize uptime,” said Marco
Arrigoni, director of marketing at
Coherent Corp.

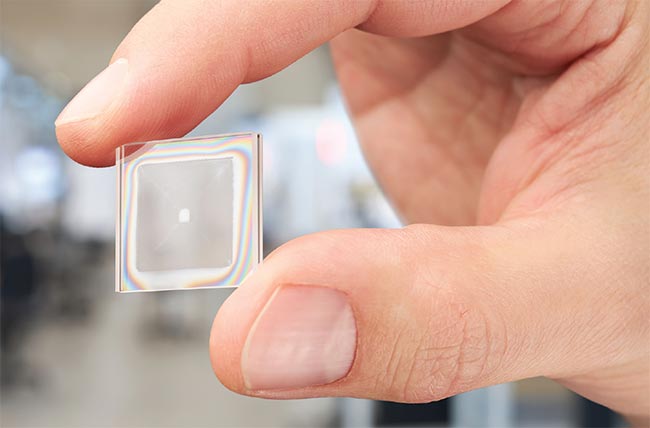
Femtosecond laser pulses enable the welding of glass to glass as well as glass to metal. Courtesy of TRUMPF.
Despite the rise in increasingly
user-friendly ultrafast systems, a better understanding of the parameters of these lasers can help to improve their throughput, quality, and efficiency in applica-
tion. Fiber-based ultrafast lasers provide
almost maintenance-free operation, lasting for many years with comparatively
affordable price points. Typical femtosecond fiber lasers with <10 W of output power, high repetition rates between 80 and 100 MHz, and pulse energies between 10 and 20 nJ cost around $50,000 — about half of the price of their early predecessors.
Cost, however, increases with average power/pulse energy. Current ultrafast
lasers can range in average power
between 10 and 200 W, with pulse widths of <300 fs, and pulse energies ranging
between 0.1 and 2 mJ with 8-mJ bursts. The price of these lasers can range
between $80,000 and $100,000.

Ultrashort-pulse lasers are finding increasing use for black marking of stainless-steel medical instruments, for which the laser energy creates an indelible mark that is less prone to corrosion and oxidation. Courtesy of NKT Photonics.
Design decisions must account for the cost versus the physical properties of
the required laser tool, said Bernhard Wolfring, product manager of Ultrafast Lasers at TOPTICA Photonics AG. “A minimum required power does not allow costs to be reduced below a certain level,” he said. “A maximum power requirement, on the other hand, helps to avoid oversizing the system in terms of both cost and capability. The result is usually a dedicated laser system with the best possible design-to-cost and design-to-parameter balance.”
Materials processing
Femtosecond lasers continue to see adoption for materials processing applications, such as cutting foils for flat panel displays, micromachining medical stents, and wafer scribing.
Compared to pico- or nanosecond pulses, femtosecond pulses achieve better quality for micromachining applications due, in part, to their minimal contribution to thermal defects, such as the heat-affected zone (HAZ) or debris around a processed area. Notably, there is a floor
to these benefits: Pulses shorter than
350 fs have not shown increased process efficiency for most materials and can require more expensive optics. Further, pulse width alone is only part of the story.


Micromachining cardiac stents is a familiar application for femtosecond lasers due to these sources' suitability for micromachining fine features. Courtesy of MKS Spectra-Physics.
“In our belief, pulse width can be a little misleading,” said Hui Imam, director of strategic marketing for Ultrafast Lasers at NKT Photonics. “The important parameter is peak power, which is the amount of energy that is imparted in the short femto space of time. The higher the peak power for a given short femto pulse, the more material is ablated with little thermal impact.”
Reducing thermal processing is critically important in materials that are temperature or mechanically sensitive, such as nitinol, polymers, drug-infused materials, or thin dielectrics.
The pulse energy and average power of ultrafast fiber lasers is limited by the damage threshold of the fiber. Amplification architectures, such as slab and disk amplifiers, can produce higher pulse energies and higher average powers. But they also come with a larger footprint, higher costs, and more stringent demands on cooling.
Average power and repetition rate determine the maximum pulse energy that a single laser pulse can achieve. For most materials processing applications, the optimal pulse energy depends on the so-called ablation threshold.
This threshold is different for different materials, but once pulse energy surpasses a material’s ablation threshold, saturation of the process can occur. In essence, the plasma created during the ablation process absorbs the subsequent pulses, adding heat and reducing processing efficiency.
“For ablation of most materials, typical pulse energies are between 0.02 to 0.2 mJ
when using femtosecond pulses,” George said.
The extremely high-power density of a femtosecond laser pulse can also induce two- or multiphoton absorption in materials to produce 3D structures with fine resolution beyond the optical diffraction limit. Compared with traditional micro/nano fabrication techniques, femtosecond laser processing provides both nanoscale feature sizes and 3D architecting capability.
The optical energy delivered per unit area — known as laser fluence — dictates the ablation rate efficiency (mm3/min/W). The optimum peak fluence value, which balances the highest machining quality with the most efficient use of the optical energy, is ~1 J/cm2 for most materials.
“Fluences below the peak result in sharply falling efficiencies, whereas higher fluences see a more gradual decline,” said Jim Bovatsek, senior applications engineering manager at MKS Spectra-Physics. “Throughput can then be scaled up by operating at higher repetition rates and hence higher average powers.”
At some point, however, either the supporting motion/scanning equipment cannot move fast enough, or the material’s ability to dissipate residual heat-energy is overwhelmed, or both, and the result is an undesirable HAZ formation.
For cutting materials such as nitinol, laser repetition rates and pulse energies of 100 kHz and ~80 µJ respectively seem to be an upper limit before HAZ formation begins, and powers >100 W at pulse rates >2 MHz are usable for cutting polymer films such as polyethylene terephthalate and polyimide, Bovatsek said.
Marking medical parts
Femtosecond lasers can be an ideal technical solution for marking reusable medical instruments, assuming the volume of production compensates for the price of the laser.
Marking a black or dark permanent 2D barcode onto a medical instrument is a growing application to register these tools and keep track of when they were cleaned.
Typically, marking these items relied on lower-cost nanosecond-pulsed lasers. The marks were chemically treated to
make them resistant to corrosion. How-
ever, femtosecond lasers create an indelible mark that is less prone to corrosion and oxidation over time, potentially removing the need for the additional chemical processing step.
Processing metals with picosecond or femtosecond lasers can create small periodic structures on the nanometer scale that appear as high-contrast black marks, said TRUMPF’s George. “These black markings are independent of the angle of observation, showing a black contrast for any angle of observation,” she said.
Research continues into whether femtosecond pulses achieve better quality marks than picosecond pulses. But higher repetition rates allow faster scanning and hence shorter cycle times. Therefore, medical marking applications must consider the trade-off of speed versus quality.
“For black marking, low pulse energies are used (<0.05 mJ) with high repetition
rates (1 MHz),” said Daniel Huerta-Murillo, a laser applications engineer at TRUMPF. “Higher pulse energies can lead to structuring of the material, and insufficient pulse energy creates a low-contrast mark.”
Welding and cleaving
Processing brittle materials such as glass is another emerging industrial market for femtosecond lasers.
According to Antonio Castelo, photonics technology manager at the European Photonics Industry Consortium (EPIC), glass processing requires a combination of the right wavelength and pulse energy. Failing to use the correct parameters often adds more time at the end of the process for extra polishing steps.
“Some of the processes used for glass and polymer materials could require different wavelengths in the NIR and mid-IR, for which there are turnkey solutions nowadays at 2 and 3 microns,” Castelo said.
Cleaving and welding of glass or transparent brittle plastics requires a special optic to achieve a certain pulse profile known as a Bessel beam. This type of beam can create a linear series of focal points through the processed material, similar to an elongated laser knife, that can modify it in a single pass.
The maximum thickness that can be processed is limited by the pulse energy. The thicker the material, the higher the pulse energy needed.
“For glass welding, pulse energies between 0.01 mJ and 0.04 mJ are used, depending on the type of material,” said TRUMPF’s Huerta-Murillo. “For glass cleaving, pulse energies ranging from
0.1 mJ and up to 2 mJ can be used, depending on the thickness of the samples
to be processed.” TRUMPF applications lab has laser-cleaved glass sheets up to
12-mm thick.
Pulse duration is another important factor in glass processing. Cleaving transparent glass, for example, can rely on picosecond pulses. But for welding transparent glass, femtosecond pulses are more useful due to the higher peak power that they can achieve, which allows the glass to melt in a very localized zone.
Multiphoton microscopy
Femtosecond pulses are particularly useful for inducing the multiphoton events that are valuable to biological and scientific imaging applications, such as nonlinear microscopy, two-photon optogenetics, and three-photon imaging.
Turnkey instruments are readily tapping into these markets, whose primary end users are biologists and neuroscientists with limited backgrounds in optics. Adding functionality to simplify the laser tool is particularly appealing to these end users.
“It always comes back to the added value for the user, most often in terms of convenience and ease of use,” Coherent’s Arrigoni said. “A core imaging lab with frequently rotating and inexperienced users may benefit from full turnkey performances and easily accept 2× the price for a turnkey laser system.”
About 80% of scientific applications for ultrafast lasers are addressed by turnkey lasers delivering 10 W of average power with pulse rates anywhere between 1 kHz
and 10 MHz, pulse widths adjustable between 20 and 200 fs, and tunable wavelengths between 200 and 1000 nm.
Pulse energy or peak power is usually the most important factor for femtosecond lasers targeting multiphoton phenomena in life sciences applications, Wolfring said. To achieve good results, these parameters must be kept within certain limits. If they are too low, the two-photon process may not be efficient enough to produce microscopy images with good contrast. If they are too high, the microscope image will probably show a burned tissue sample.
In general, multiphoton imaging applications require lasers to deliver tens to hundreds of nanojoules between 1 and 100 MHz pulse rates to support fast image scanning and to avoid damage to the biological sample.
In principle, the shorter the pulses, the higher the nonlinear effect, though maintaining the short pulse widths as they travel through the optical system is an important factor; parameters such as dispersion and compensation effects become very important.
Balancing idealistic with realistic
Ultrafast lasers are still finding new
applications in fields ranging from spectroscopy to photonics computing.
Customers in these sectors demand flexibility and tunabilty from lasers, but the lifetime of ultrafast sources does not yet match the scale of the market. Florian Emaury, CEO at Menhir Photonics,
believes that finding the trade-offs to supply these emerging markets is a challenge worth tackling.
The design of an ultrafast laser system for these markets must balance customer needs with a reasonable delivery in terms of reliability and manufacturability. Building a robust turnkey system requires iterative steps — the first is to identify the minimal specifications required for the desired application.
Customer demands are always arguably valid for their experiments, Emaury said, but customers rarely account for the aspects of reliability and reproducibility that their desired system will require. “Thinking about the ownership cost of the laser over many years is key,” he added.
Footprint, fiber, and the future
In addition to becoming easier to use and more competitively priced, ultrafast lasers are becoming more compact. Fiber-based systems allow more flexible delivery of the beam, which can facilitate integration in a cramped production line, a microscopy system, or a medical office.
Smaller footprints also allow ultrafast lasers to fit into smaller devices, for example photonic computing architectures, in which the precision of ultrashort pulses might enable photonic microprocessors to perform calculations more quickly and with less energy.
“We see it as a very high-demand market for which we plan to produce hundreds of thousands to even millions of lasers per year,” said Emaury. “Of course, these lasers will be smaller than the size of a cellphone at this point, but they will become the center part of any high-end computer.”
Lasers specified for photonics computing will need to deliver repetition rates in the GHz and precisely time each pulse within a 10-fs window.
While this and other markets might require sophisticated parameters that cannot yet be achieved with today’s lasers, their recent progress provides impetus for new developments.
As ultrafast lasers evolve, more powerful and versatile performance in terms of repetition rate, wavelength, and pulse duration will inevitably cause their adoption to increase. But there seems to be something of a give and take in how technology drives markets and vice versa.
“The evolution of fiber laser developments in recent years shows that new laser wavelengths and new power levels — achievable through new concepts such as chirped pulse amplification — have given fiber lasers a major technology push to enter their current key markets,” Wolfring said.