Colloidal quantum dots offer a scalable, cost-effective alternative to traditional SWIR sensing.
SAM WYMAN, ALLAN HILTON, AND ETHAN KLEM, SWIR VISION SYSTEMS
In the realm of advanced imaging, shortwave infrared (SWIR) technology has undergone an identity challenge for more than 20 years. Typing “SWIR imagery” into a search engine often results in the same examples: visible images juxtaposed with SWIR ones, highlighting its ability to detect bruises on apples, ascertain fill levels in opaque shampoo bottles, and pierce through foggy coastlines.
Despite SWIR’s unmatched proficiency in these and other use cases in visually obscured environments, it has not reached widespread adoption. The main hurdle? Cost. The field has traditionally been dominated by indium gallium arsenide (InGaAs) sensors, which, despite their efficacy, are expensive and not easily scalable, confining their use to sectors in which cost is a secondary concern, such as the military and space industries.
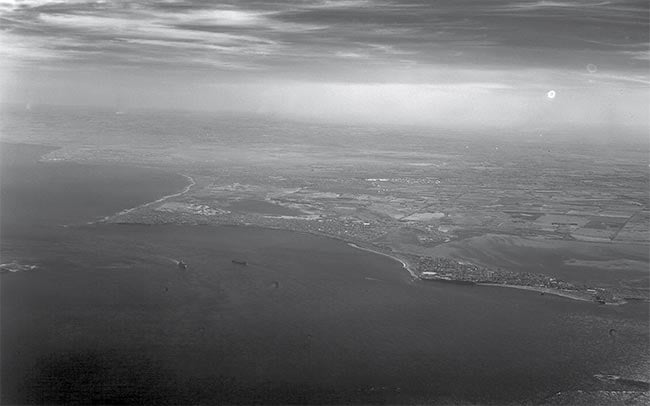
An aerial image through maritime haze and clouds. Courtesy of SWIR Vision Systems.
Enter colloidal quantum dot (CQD) technology, an innovative approach
that makes SWIR sensing more accessible and cost-effective. At the heart of this innovation is the use of nanoscale semiconductor particles, which can be precisely engineered to absorb and emit light at specific wavelengths, including the SWIR range. This article sets out to explore CQD SWIR technology from its conceptual beginnings through its developmental challenges to its current adoption and beyond. The authors delve into how this technology, with its scalable, wafer-level sensor fabrication approach, is not only making SWIR sensors more affordable but also broadening their application horizons. The authors examine the early development, the state-of-the-art advancements, and the promising future that lies ahead in redefining
IR sensing.
The advent of CQD SWIR
To fully leverage the capabilities of CQD SWIR sensors, it is crucial to consider the entire imaging system, including optics, illumination, and filtering.
The development of CQD SWIR technology began with a vision to overcome the constraints imposed by traditional InGaAs sensors, primarily their prohibitive costs and scalability issues. Early research into CQD materials showed promise due to their unique optical properties, which could be tuned to the SWIR range by adjusting the size of the quantum dots.
One of the primary difficulties was fabricating high-quality quantum dot thin films that had sufficient charge transport properties to enable the efficient extraction of photoexcited carriers. They had to be produced without resulting in dark current densities that are significantly higher than the 1 to 10 nA/cm2 range that is typically exhibited by lattice-matched InGaAs detector arrays. One of the primary processes that affected these properties was optimizing dot surface passivation to reduce carrier recombination and increase carrier mobility.
Another challenge was integrating these quantum dots into a functional sensor architecture. Traditional sensor fabrication of a SWIR InGaAs detector used pixel-level, metal-to-metal hybridization to attach an array of SWIR-sensitive photodiodes fabricated on an indium phosphide wafer to the surface of a readout integrated circuit (ROIC) fabricated in silicon.
In contrast, CQD detectors are thin-film devices using amorphous materials. CQDs are typically spin coated directly onto a silicon ROIC wafer with additional layers of device stack being deposited on top through methods that include evaporation, sputtering, and other common thin-film deposition techniques. The fabrication of a CQD image sensor required the development of transparent conducting oxides, charge blocking layers, and encapsulation layers that could all be deposited over the top of CQD layers without negatively affecting the electro-optical properties of the CQD layer.
Breakthroughs in CQD technology
Despite these obstacles, persistent research and development led to significant breakthroughs. Advancements in chemical synthesis methods allowed for the production of quantum dots with the desired optical properties and stability. Researchers such as Ethan Klem, CTO of SWIR Vision Systems, developed new photodiode structures for producing quantum dot photodetectors on sensor substrates. Examples of these structures included PN junctions as well as Schottky junctions formed between CQDs and metal films, such as aluminum and magnesium.
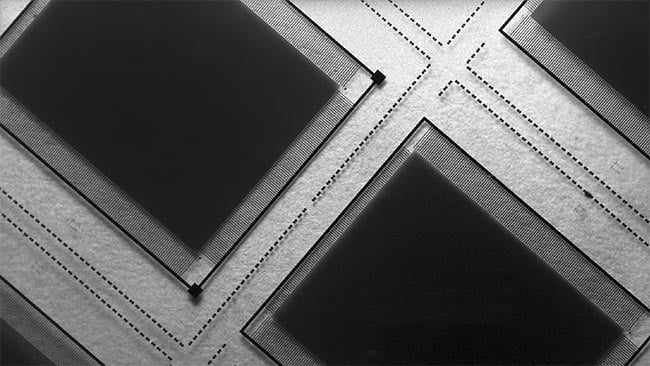
An image of a silicon wafer taken with an Acuros 2-MP SWIR camera. Courtesy of SWIR Vision Systems.
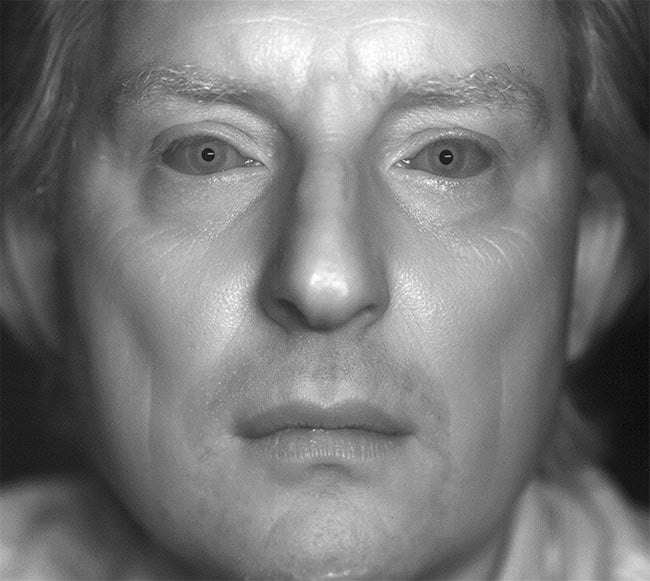
A portrait of SWIR Vision CTO Ethan Klem. Courtesy of SWIR Vision Systems.
A pivotal advancement emerged with the development of a scalable, wafer-level fabrication process. This process enables the mass production of CQD SWIR sensors, significantly reducing costs and making the technology viable for a broader range of applications. For example, a single 200-mm wafer has ~250 die per wafer (assuming a die size of 10 × 10 mm). In contrast, processing singulated die results in a capacity of ~20 die per day on an equivalent set of processing equipment. The ability to fabricate these sensors on standard silicon substrates further enhanced their compatibility with existing manufacturing infrastructures, paving the way for widespread adoption.
As the technology matured, the focus shifted toward optimizing the performance and reliability of CQD SWIR sensors. Efforts to enhance quantum efficiency (QE), reduce noise, and improve the dynamic range have steadily pushed the boundaries of what these innovative sensors can achieve. For example, dark currents of CQD detectors are typically <10 nA/cm2. This has decreased by a factor of 20 or so compared to earlier work. QE, on the other hand, is frequently reported to be >50%, a significant advancement from the sub-1% QEs observed from the first CQD SWIR photodiodes.
CQD SWIR sensor fabrication
The fabrication of CQD SWIR sensors represents a significant departure from traditional sensor manufacturing processes, primarily due to the novel properties and requirements of CQDs. This section delves into the scalable, wafer-level approach that has been pivotal in making CQD technology a viable and competitive alternative in the infrared sensing market.
At the core of the CQD SWIR sensor’s appeal is its scalable, wafer-level fabrication process. This method produces sensors on a scale and at a cost that was previously unattainable with InGaAs-based sensors. The process begins with the deposition of a thin film of quantum dots onto a substrate, typically silicon, using processes commonly used throughout the silicon semiconductor industry. This process compatibility is crucial because it enables CQD sensor manufacturers to leverage existing semiconductor fabrication infrastructure, significantly reducing the cost and complexity of sensor production.
Compatibility with ROIC designs
A feature of CQD SWIR photodiodes developed by many researchers is their compatibility with standard ROICs developed for InGaAs and other infrared photodiode technologies. This compatibility arises from similar dark current densities and capacitance of CQD photodiodes as compared to traditional IR detector materials. The similarities in electrical characteristics have resulted in CQDs being integrated with standard ROIC front-end amplifier designs, such as capacitive transimpedance, direct input, and source follower. This means that CQD focal plane arrays can leverage existing ROIC designs, reducing cost and time to market.
This ROIC agnosticism is enabled by the flexibility of the CQD material system, which can be applied to different ROIC platforms without compromising the detector’s performance. This adaptability extends the potential applications of CQD SWIR sensors beyond traditional defense and industrial markets, making them suitable for a broader array of industries and uses.
The combination of scalable fabrication and ROIC compatibility underscores the transformative potential of CQD SWIR technology. By offering high-resolution, cost-effective sensors that can be customized for diverse applications, CQD technology is set to redefine the landscape of infrared sensing.
Advancements and reliability
As CQD SWIR technology matured, significant strides were made not only in its performance but also in its reliability and high-temperature stability, making it suitable for a wider range of applications, from automotive to aerospace and beyond. This section highlights the key advancements that have contributed to the enhanced reliability of CQD SWIR sensors and their ability to meet the rigorous demands of various industries.
One of the most critical developments in the evolution of CQD SWIR sensors has been the significant improvement in their reliability. Early versions of CQD sensors faced challenges related to environmental stability, particularly under conditions of high temperature and humidity. Through dedicated research and refinement of the quantum dot materials and sensor architecture, these issues have been largely overcome.
Today’s CQD SWIR sensors have been designed to pass harsh environmental stress tests required for qualification in automotive and defense applications. Examples include 1000 h of 85 °C/85% relative humidity exposure, 1000 h of 125 °C exposure, and 1000 cycles of −40 to 125 °C thermal cycling. This robustness is a testament to the advancements in the protective coatings and encapsulation techniques used in CQD sensor fabrication, which safeguard the quantum dots from degradation via atmospheric ingress.
Radiation testing
The reliability of CQD SWIR sensors under extreme conditions extends
to their performance in radiation-
rich environments, making them suitable for space applications. Initial results from radiation exposure testing carried out in U.S. government labs have demonstrated that CQD sensors can withstand the levels of ionizing radiation encountered in orbit without a change in performance. This resilience enables new possibilities for satellite and space exploration missions, in which reliable SWIR sensing can provide critical data for Earth observation, astronomical research, and planetary exploration.
The advancements in the reliability and robustness of CQD SWIR sensors have significantly broadened their application horizons. Beyond traditional imaging and surveillance, these sensors are being explored for use in autonomous vehicles, in which their ability to perform in diverse environmental conditions can contribute to safer navigation and obstacle detection. CQD SWIR sensors can produce high-resolution reflected light 2D images that can be processed with machine vision hardware and software developed for visible image sensors.
The use of SWIR in automotive will be complementary to lidar and other sensor approaches. Particularly in conditions of rain, smoke, haze, and snow. In the consumer electronics sector, the potential for integrating CQD SWIR sensors into everyday devices promises to unlock new functionalities and enhance user experiences.
As the technology continues to evolve, its influence is set to permeate various industries, bringing the benefits of SWIR sensing to industries and applications that were previously beyond reach.
Future prospects
The evolution of CQD SWIR technology has arrived at a crucial crossroads, showcasing capabilities that not only rival but, in some cases, surpass traditional benchmarks in infrared sensing. This segment provides an overview of the current advancements and challenges within CQD SWIR sensors, while also looking forward to the potential developments on the horizon.
QE is a pivotal metric in assessing a sensor’s capability to transform incident photons into electrical signals. Presently, CQD SWIR sensors are known to have lower QE in comparison to their InGaAs counterparts. This area remains a hotbed for research, with efforts concentrated on refining the materials used in quantum dots and enhancing sensor architectures to boost light absorption and the mobility of charge carriers.
However, it is critical to reframe the conversation around sensor performance from a focus on QE to a broader evaluation of signal-to-noise ratio (SNR). SNR is increasingly recognized as a more comprehensive indicator of image quality, encompassing not only the QE of pixels but also factors such as pixel size, the efficiency of the optical system, and various noise components, including detector and readout circuitry noise.
In the context of comparing various sensors, the pertinent question shifts from “What is the QE?” to “What is the SNR?” This transition underscores the importance of SNR in providing a fuller picture of image quality and the richness of information within image data.
Despite the QE challenges, the unique attributes of CQD technology, notably its cost-effectiveness and scalability, continue to support its integration into various applications. While the trade-off between QE and these advantages is a critical consideration for specific applications, ongoing advancements in the field are progressively mitigating this gap, signaling a promising trajectory for the future of SWIR imaging.
State-of-the-art resolution
The resolution of SWIR sensors is a critical factor in many applications, from high-definition imaging to detailed spectroscopic analysis. CQD SWIR technology has achieved a significant milestone with sensors reaching
resolutions of up to 1920 × 1080 pixels and pixel pitches as small as 3 µm. This level of resolution represents a considerable achievement, providing high-quality imaging capabilities for a wide range of applications.
To fully leverage the capabilities of CQD SWIR sensors, it is crucial to consider the entire imaging system, including optics, illumination, and filtering. The integration of SWIR-optimized optics is essential for maximizing the sensor’s performance, ensuring that the SWIR light is efficiently captured and focused onto the sensor array.
Active illumination sources, such as fiber lasers and SWIR LEDs, play a vital role in enhancing image quality under varying lighting conditions.
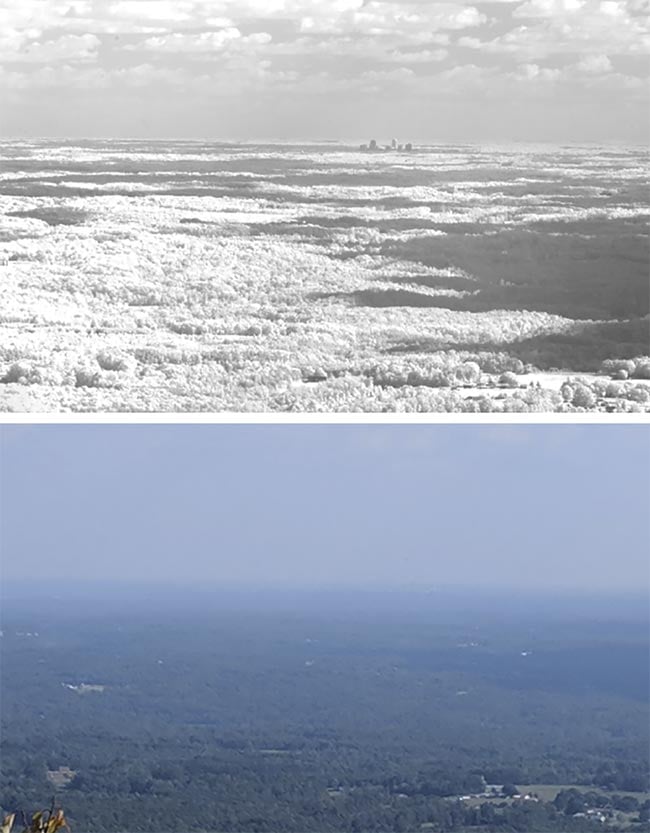
A comparison of a mountainside in Winston-Salem, N.C. A SWIR image (top) and a Google Pixel 4 image (bottom). Courtesy of SWIR Vision Systems.
Customized filters are another key component, allowing for the selective transmission of SWIR wavelengths to meet the specific requirements of different applications. These system-level considerations are critical for optimizing the performance of CQD SWIR sensors and unlocking their full potential.
The future landscape
As CQD SWIR technology continues to evolve, its influence is poised to
expand across various sectors, notably in defense, in which its potential applications are vast and critical.
In the defense sector, the strategic importance of advanced sensing
capabilities is critical. CQD SWIR
sensors, with their enhanced resolution, robustness, and reliability, are ideally suited for surveillance and reconnaissance to target acquisition and night vision using advancements in active SWIR illumination. The technology’s ability to perform in adverse conditions — such as low light, fog, and smoke — makes it suitable for operations in challenging environments.
Beyond the defense sector, the automotive and consumer electronics sectors are among the most promising areas for the adoption of CQD SWIR technology.
The automotive industry will benefit from the enhanced sensing capabilities of CQD SWIR sensors in the development of autonomous vehicles, improving safety and navigation through superior obstacle detection. In consumer electronics, the integration of CQD SWIR sensors into devices such as smartphones and wearables could improve features such as night vision and health monitoring, enriching user experiences.
Vision for the future
The future of CQD SWIR technology promises to redefine the capabilities of infrared sensing across a spectrum of applications, from defense and automotive to consumer electronics and beyond. The focus on enhancing sensor performance, combined with efforts to integrate these sensors into optimized systems, is set to maximize the potential of SWIR sensing. Additionally, the environmental and economic advantages of CQD technology, such as lower energy requirements and reduced manufacturing costs, align with global sustainability goals, further bolstering its appeal.
The advancements in CQD SWIR technology mark a significant milestone in the field of infrared sensing, with far-reaching implications across various industries, including defense. As the technology progresses, it promises to not only enhance existing applications but also to open new frontiers in sensing capabilities. From its early challenges to its future prospects, the evolution of CQD SWIR technology exemplifies the transformative power of innovation, heralding a new era in which advanced sensing technologies play a pivotal role in shaping the future of defense and beyond.
Meet the authors
Sam Wyman is global sales manager at SWIR Vision Systems and brings more than a decade of expertise in the photonics industry, specializing in advanced optical IR systems, sensors, and cameras. His leadership drives the global expansion of SWIR Vision Systems’ cutting-edge technologies; email: [email protected].
Ethan Klem is CTO and cofounder
of SWIR Vision Systems and focuses on advancing CQD detector technology and applications. He holds a doctorate
in electrical engineering from the University of Toronto and has worked on the development of CQD-based optoelectronics devices for more than 20 years; email: eklem@swirvision
systems.com.
Allan Hilton is chief product officer and cofounder of SWIR Vision Systems and oversees the development of
CQD SWIR imaging solutions. He
holds a bachelor’s degree in materials science from North Carolina State University and has more than two decades of experience developing and deploying
new semiconductor technologies; email: [email protected].
The trajectory of CQD SWIR sensors mirrors that of CMOS sensors, which have become ubiquitous due to their scalability, performance, and cost-effectiveness. As CQD technology advances, particularly with improvements in quantum efficiency (QE) and the introduction of higher-resolution models, it is expected to achieve a level of adoption and influence comparable to that of CMOS sensors, making advanced SWIR sensing accessible to a wider range of applications.