Despite tremendous accomplishments in recent years, superresolution microscopy is a relatively young field, and confusion exists in this domain of many abbreviations. However, this makes for captivating times as superresolution microscopy opens the door to wider imaging applications.
Marie Freebody, Contributing Editor
Superresolution optical microscopy, for which the Nobel Prize was awarded to Eric Betzig, Stefan Hell, and William Moerner in 2014, has been one of the most momentous developments in the life sciences over the last decade — and its evolution is ongoing. Progress has been so rapid and significant that it has been dubbed by some observers as “the resolution revolution.”
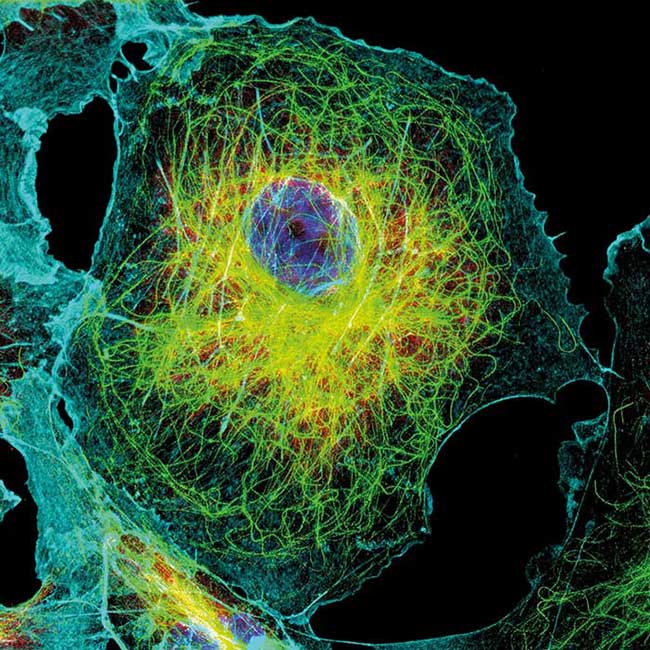
Multicolor STED image — vimentin, Alexa 647 (red); alpha-tubulin, Alexa 594 (green); F-actin, Alexa 488 phalloidin (cyan). Confocal, DAPI (blue). Courtesy of Eugene Katrukha/Utrecht University.
The demands from biomedical applications are very wide — ranging from
the need for high spatial and temporal resolution, long acquisition
time, 3D imaging, deep tissue/in vivo imaging, and so on. Far-field
optical microscopy using focused light is an important tool,
particularly for studying living cells and organisms. Unfortunately, the
optical microscope is limited; the diffraction of light imposes
limitations on the spatial resolution of the image.
This means that the details of cellular protein distributions, for example, can be visualized only to a certain extent. Fortunately, recent years have witnessed the development of superresolution far-field optical microscopy (nanoscopy) techniques such as STED, RESOLFT, PALM, STORM, GSDIM, SIM or SSIM, and FINCH/CINCH (see end of article for acronym definitions), all in one way or another addressing the problem of limited spatial resolution of far-field optical microscopy.
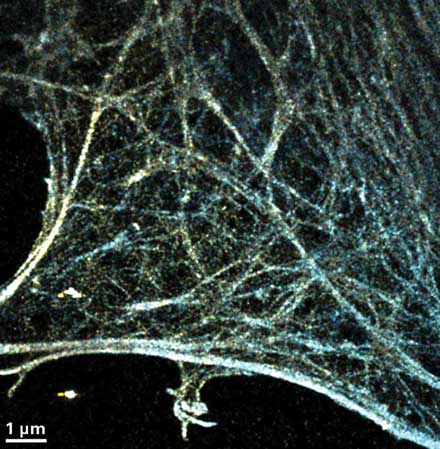
Actin cytoskeleton in part of a cell, imaged using 3D-STORM. The projection image has been color-coded for depth. The actin cytoskeleton was marked with a dye-labeled, small nonantibody binding protein, specific for actin. Courtesy of Ruth Hughes/Alistair Curd/University of Leeds.
SIM achieves an incredible twofold improvement in spatial resolution
compared to conventional optical microscopy, but STED, RESOLFT,
PALM/STORM, and SSIM have gone even further, pushing the limits of
optical image resolution to the nanometer scale. Consequently, all
superresolution techniques open new avenues of biomedical research.
“In principle, any of these techniques can be applied to any sample to provide a ‘superresolved’ image,” said professor Michelle Peckham from the Astbury Centre at the University of Leeds, England. “In practice, individual choices will include the availability of dyes, probes, antibodies or fluorescent protein tags available to image the protein of choice, alongside equipment availability and ease of use.”
SIM: Complex, but a good compromise
“SIM applies structured illumination to achieve an improvement of a factor of two over conventional microscopy — no photoswitching or blinking as in STED/RESOLFT and PALM/STORM is needed,” said professor Christian Eggeling, principal investigator and scientific director of the Wolfson Imaging Center Oxford, MRC Human Immunology Unit at the University of Oxford, England as well as Professor for Super-resolution Microscopy at the Friedrich-Schiller University and Leibniz Institute of Photonic Technologies, Jena, Germany. “Therefore, SIM can be applied to conventional samples, fixed or living cell, and even in 3D.”
For many biological imaging applications a factor of two is good enough, making SIM a good compromise between spatial resolution and long acquisition times. But there are disadvantages.
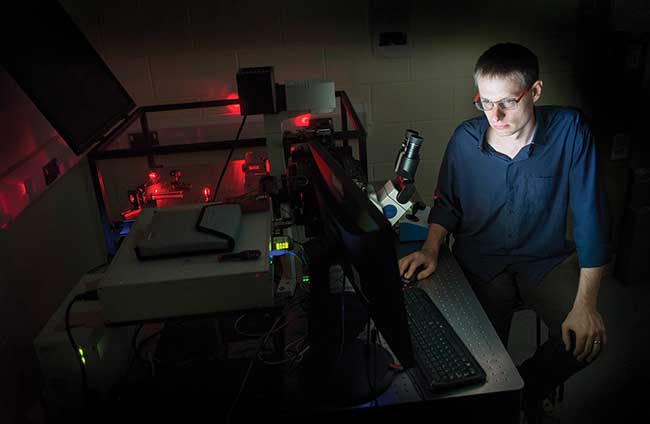
Alistair Curd, a researcher in the Peckham group working on the 3D STORM/PALM setup, at the University of Leeds Astbury Center. Courtesy of Mark Bickerdike/University of Leeds.
A disadvantage is that several images need to be recorded for every one final image, which prolongs total acquisition time and thus reduces temporal resolution, especially for 3D recordings. Still, the recording time of SIM has steadily improved, and more and more related techniques such as rescan or Airy-scan microscopy have emerged, which only require one image per final image. In particular, iSIM acquires single images very rapidly, making it useful for imaging moving targets within cells. In general, SIM and related techniques are a good compromise and possibly the best technique for nuclear/chromosomal imaging.
FINCH/CINCH
A holographic approach to superresolution, FINCH can also create the superresolved image in one snap and is simpler, and less expensive than some approaches. Since its invention at CellOptic, Inc. in Rockville, MD, it has been under development for more than a decade by Dr. Gary Brooker, CEO of CellOptic. In collaboration with Dr. Nisan Siegel at the company, they have created a confocal microscope called CINCH that produces a confocal superresolution image in a single exposure with 100-nm resolution. The all-solid-state configuration is readily adapted to existing microscopes.
FINCH was the first and simplest form of incoherent holography. Now with a single birefringent lens, FINCH creates holograms from an object emitting incoherent light in a single-beam single-snap system by self-interference between two spherical waves originating from the object. Light originating from any point in the object is split into two beams that are differentially phase-modulated and recombined in a common plane to produce interference fringes. A report published in Nature Photonics (doi: 10.1038/nphoton.2016.207) highlighted the ability of FINCH microscopy to rapidly produce superresolved biological images in multiple colors.
“FINCH stands out from other superresolution methods in its simplicity and ease of use, requiring only a specialized imaging lens and fast calculation without requiring extensive illumination strategies, extremely large data sets, or limited staining options,” Brooker said. “FINCH can provide resolution similar to SIM at speeds that enable live-cell imaging for almost any microscopic application.”
STED: You get what you see
Unlike SIM, STED gives a direct image without the need for data analysis. As well as being straightforward, STED is rather versatile, making it suitable for fixed and live cells. In principle, this utilizes the typical labeling dyes used for confocal/wide-field microscopy. In practice, STED-specific dyes are generally used.
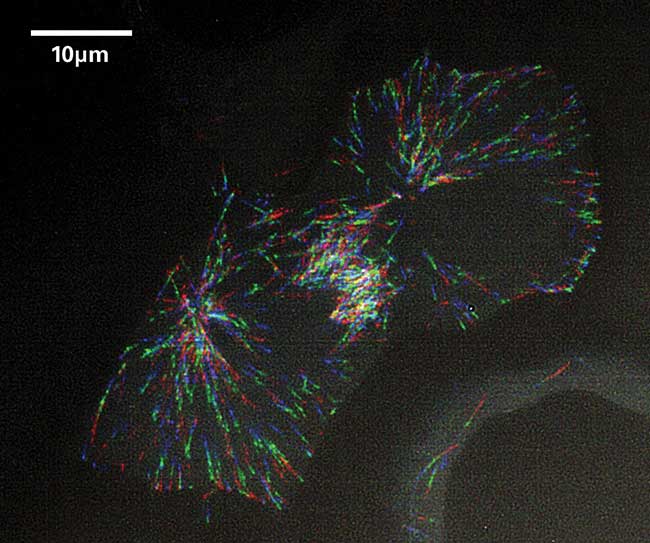
Image of eGFP-EB1 in live cells, collected using the iSIM microscope. This is a summary image of a time-lapse sequence, where sequential positions of eGFP-EB1 are color-coded (representing change in position with time). Courtesy of Ruth Hughes/Alistair Curd/Darren Tomlinson/Anna Lopata/Christian Tiede/Michelle Peckham.
“The STED technique enables the optical imaging of subcellular structures with nanoscale resolution. Multiphoton-excited fluorescence imaging offers intrinsic optical sectioning, limited photobleaching and phototoxicity, and deeper penetration, all key advantages for imaging in scattering tissue,” said Julien Klein, senior manager of product marketing for Spectra-Physics.
Spectra-Physics supplies ultrafast lasers to enable hybrid STED microscopes that combine the benefits of superresolution and multiphoton-excited (MPE) fluorescence into one setup.
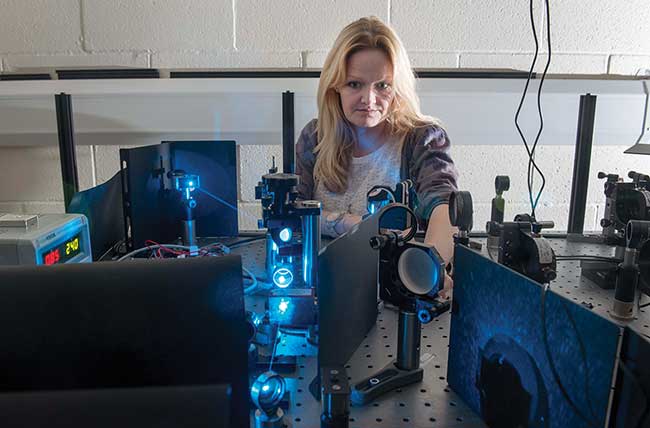
Researcher Alexa Cleasby, a Wellcome Trust ISSF-funded research fellow, checks the alignment of optics on the iSIM superresolution microscope built by the Peckham group at the University of Leeds Astbury Center. Courtesy of Mark Bickerdike/University of Leeds.
“In a particular scheme, one can simultaneously obtain the advantages of STED and MPE imaging by combining a femtosecond infrared 1-µm laser, such as Spectra-Physics’ HighQ-2, for two-photon excitation and a visible CW laser for depletion,” Klein said.
A unique feature of STED is that the spatial resolution can be tuned with the intensity of the added STED laser — both in 2D and 3D, and from confocal down to in-principle unlimited scales. This gives greater flexibility in image acquisition.
“For example, if the sample is not too bright or sample preparation is not too accurate one might sacrifice spatial resolution for better signal-to-noise,” Eggeling said. “On the other hand, the tuning of the spatial resolution allows unique applications such as STED-FCS [fluorescence correlation spectroscopy on a STED microscope] for revealing diffusion modes of molecules with unprecedented detail.”
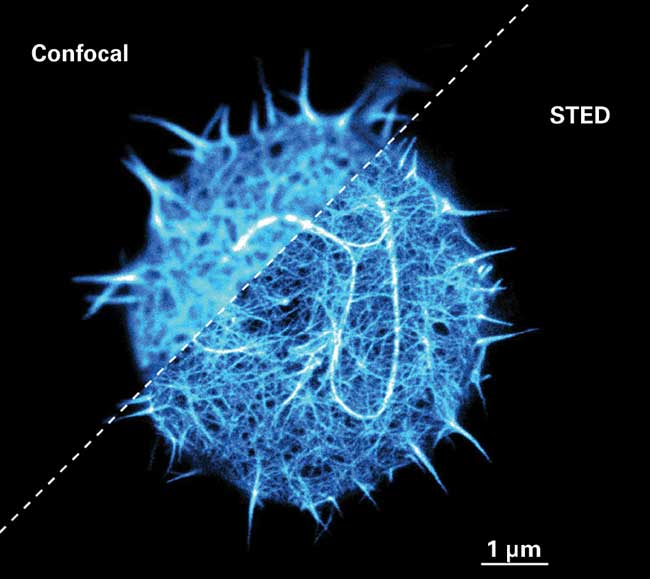
Confocal and STED microscopy image of F-actin (Lifeact-Citrine) in a live Jurkat T-cell. This data was taken by Mathias Clausen and Marco Fritzsche. Courtesy of Weatherall Institute of Molecular Medicine, University of Oxford.
STED microscopy is usually considered very phototoxic, both with respect
to photobleaching of the labels and cell viability, due to the high
laser intensities involved. However, more and more live-cell application
reveals STED microscopy as an appropriate tool for observing cellular
dynamics with high spatial and temporal resolution, if great care is
taken in the acquisition mode, labeling protocol, and sample preparation.
Ultrafast lasers also may be used in multiple schemes, such as creating a two-photon-excitation pulse train or a high-repetition-rate chirped depletion beam. For example, a near-IR laser, such as the Spectra-Physics Mai Tai, can produce a high repetition rate pulsed depletion beam for use in STED by stretching the pulse duration to hundreds of picoseconds through a glass rod and/or an optical fiber.
COS-7 cells acquired with HyVolution 2. Courtesy of Jana Doehner/University of Zurich Center of Microscopy and Image Analysis.
Leica Microsystems’ Rolf Borlinghaus describes STED as the best approach
for investigating thicker samples that contain a true third dimension.
With the superresolution technology LIGHTNING and the Leica TCS SP8 STED Nanoscope, Leica Microsystems aims to help scientists in
neurobiology, cancer research, and immunology to visualize molecular
interactions and structures in living cells, in order to understand
life’s true nature.
Finally, a new STED instrument is being developed by Abberior, including the STED attachment for widefield microscopes (STEDYCON), together with the cutting-edge approach of MINIFLUX that promises resolutions of up to 1 nm.
Single molecule localization microscopy
PALM/STORM/GSDIM: These are different acronyms, but each approach points to the same basic principle. Their popularity is down to a relatively easy and cost-efficient setup since they can comprise simply of a standard total internal reflection fluorescence (TIRF) /wide-field microscope with a sensitive camera. They also typically achieve the highest spatial resolution in common experiments, and labeling can be done with conventional dyes and special buffers to make them blink, or with special photoactivatable or photoswitchable fluorophores.
First reported in 2006, PALM/STORM/GSDIM uses photo-induced fluorescence switching to temporally separate the spatially overlapping images of single molecules. This allows their positions to be determined with high precision and an image with sub-diffraction-limit resolution to be reconstructed from these molecular positions.
“The resolution of PALM/STORM/GSDIM is thus not limited by diffraction, but rather by the precision with which the molecular positions can be measured and, in some cases, also by the density of the localized molecules,” Eggeling said.
The disadvantages include rather tedious data analysis, hundreds to thousands of individual camera images need to be recorded to create a final superresolved image — i.e., image acquisition is quite long and observations of faster live-cell dynamics are therefore difficult; and low background noise is needed since single isolated molecules need to be switched on and off and localized, thus TIRF is still the preferred acquisition mode.
Still, this technique is steadily improving, specifically with respect to automated, unbiased data analysis, speed, and deeper-sample imaging (e.g., through using light-sheet). New software approaches, e.g. HAWK analysis, can also help reduce potential artifacts in the final images. A recent approach termed MINIFLUX — where the localization precision is improved by using features from the STED approach, resulting in the requirement of much lower photon numbers — will open up new avenues, Eggeling said. MINIFLUX works by triangulating the position of a single fluorescent molecule with different positions of an excitation pattern, allowing a precision of localization beyond typical PALM/STORM/GSDIM systems that use wide-field/TIRF illumination.
RESOLFT: Gentle illumination favors live-cell imaging
RESOLFT applies the same principles as STED, but it also uses specifically reversible, photoswitchable fluorescent labels, as for PALM. Thanks to the use of these labels, superresolution can be achieved with much lower laser intensities than in STED. The gentle illumination allows for long-term recording of neuronal proteins and their dynamics in cells and tissues at the nanoscale.
“Unfortunately the general applicability has so far been hampered by the limited availability of reversible photoswitchable fluorescent labels,” Eggeling said. “But these are constantly improved and becoming more feasible.”
SSIM: Limited application
SSIM uses reversible photoswitching on top of SIM to achieve an improvement in spatial resolution better than a factor of two. But the problems are the same as for SIM and for RESOLFT — other than proof-of-principle, the applicability remains limited.
CSREM or CLEM: A combination of electron and light microscopy
CSREM or CLEM combines electron microscopy with other superresolution techniques, usually STORM or PALM. It can be applied only to fixed samples and with markers for identifying certain sample areas, either with gold beads or marks in the cover glass.
University of Leeds’ Peckham said: “This [technique] may be useful in using superresolution imaging of live cells — to examine the behavior of a particular protein with high resolution, followed by rapid fixation (e.g., by high-pressure freezing) and imaging with electron microscopy to investigate the protein in the context of the cellular ultrastructure.”
The picture today and tomorrow
STED, RESOLFT, PALM, and STORM are now routinely used in a growing number of labs to probe transparent matter at length scales a tiny fraction of the wavelength of the imaging light. Some of the applications of these techniques are yielding information about membrane nanostructure and neuroscience and for the study of the mechanisms associated with protein aggregation linked to Alzheimer’s and Huntington’s diseases.
But there is a price to pay for gaining more spatial resolution — accurate sample preparations and optimized labels become critical. In particular, the large size of conventional antibodies starts to become limiting, with more people using nanobodies, or artificial binding proteins such as Affimers, which are smaller.
“In general, with superresolution microscopy, one needs to prepare a sample with great care. Improper labeling such as high background or improper fixation might be forgiven in confocal/wide-field microscopy, but will significantly deteriorate the superresolution images,” Eggeling said. “Similarly, if cells are not happy they will usually die faster in superresolution images compared to conventional confocal/wide-field microscopy images.”
There are techniques that try to provide compromises between improvement in spatial resolution and complexity of setup or analysis. Airy-scan or iSIM microscopy offered by several companies are both similar to SIM and achieve up to 1.7-fold increase in spatial resolution with a small addition to a conventional confocal or wide-field microscope. On the other hand, continuous efforts of organic chemists to improve the photon yield of the fluorescent labels are basis for further improvements.
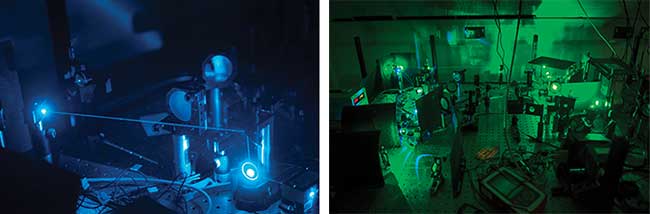
A glimpse into the iSIM, demonstrating the complex optical light path. Courtesy of Mark Bickerdike/University of Leeds.
According to the 2015 Super-Resolution Microscopy Roadmap, published by the Institute of Physics in its Journal of Physics D: Applied Physics (doi: 10.1088/0022-3727/48/44/443001), the next level of development will be to extend application of superresolution microscopy to a wider range of scientific questions. In one sense, this means that the microscopes should be capable of working with a wider range of specimens.
The Roadmap, which was co-authored by some of the biggest names in the field, including Stefan Hell and Eggeling, emphasizes that employing adaptive optics to access thicker specimens will permit more use of superresolution in tissue applications, with significant potential benefit in developmental biology, neuroscience, and other areas.
Another advantage of the wider use of adaptive optics will be increased usability of superresolution as it provides the opportunity for self-calibration and auto-alignment of systems, thus reducing the need for in-house technical support. Advances such as these are set to transform these methods into commonplace lab tools.
Deciphering the Acronyms Associated with Superresolution Microscopy
Superresolution microscopy is the umbrella term for several techniques that go beyond the diffraction limit, most of them to the nanometer scale. Some examples include:
• SIM: structured illumination microscopy
• iSIM: instant structured illumination microscopy
• FINCH/CINCH: Fresnel incoherent correlation holography, CINCH is the confocal version.
• STED: stimulated emission depletion microscopy
• PALM/STORM/GSDIM: photo-activated localization microscopy/stochastic optical reconstruction microscopy/ground state depletion microscopy followed by individual molecule return
• HAWK analysis: Haar wavelet kernel analysis
• MINIFLUX: minimal photon fluxes
• ModLoc: modulation localization
• RESOLFT: reversible saturable optical linear fluorescence transitions
• SSIM: saturated structured illumination microscopy
• CSREM/CLEM: correlative superresolution optical and electron microscopy/correlative light and electron microscopy