With the development of patterned alternating dispersion waveguides, supercontinuum sources can be made significantly more compact and efficient in a package that fits in the palm of a hand.
HAIDER ZIA SUPERLIGHT PHOTONICS, AND JAROSLAW SPERLING MENLO SYSTEMS
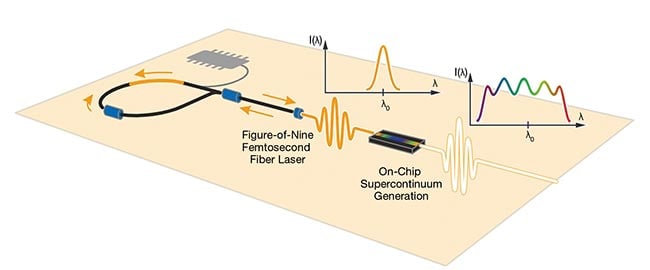
A figure-of-nine femtosecond fiber laser seeds a novel dispersion patterned chip to enable compact, low-power supercontinuum generation. The new technology could support more portable supercontinuum sources for sensing, ranging, and imaging applications. Courtesy of Menlo Systems.
Supercontinuum generation (SCG) converts laser light, typically from a pulsed laser, into coherent light that is characterized by a superwide continuous optical spectrum (Figure 1). The huge spectral bandwidths that these sources enable often span more than two octaves, making these sources instrumental in a plethora of applications.

Figure 1. The characteristic shape of a supercontinuum in the visible spectrum. It was generated with femtosecond pulses in the energy range of several nanojoules per pulse. Courtesy of Menlo Systems.
In time-resolved spectroscopy, for example, the multiwavelength characteristics of supercontinuum lasers allow for the detection of several species simultaneously. SCG is also advancing applications such as nonlinear microscopy, optical frequency combs, and hyperspectral lidar. It is not surprising that the pursuit of a more application-tailored and accessible generation of supercontinua has emerged as a field of intense research.
Yet, two characteristics of SCG technology have hampered its ability to penetrate high-volume industrial markets, which often demand ever smaller, more cost-efficient devices.
Both demands are related to efficiency, and conventional SCG sources are typically inefficient. That is, relatively large amounts of laser energy are required to achieve SCG, which drives costs up and, in practice, translates into tabletop-sized SCG devices. Further, although SCG provides a large overall bandwidth, the so-called high-spectral-power range, where spectral power is concentrated, is typically quite narrow. A broader high-spectral-power range, also referred to as 1/e bandwidth, is crucial for interferometric applications such as OCT. In most cases of conventional SCG, however, the 1/e bandwidth will be only slightly wider than that of the seed laser itself.
To overcome these constraints, major industrial players in the OCT, lidar, and other laser-based sensing markets have been turning to scanning-continuous-wave lasers that provide a large high-spectral-power range (via scanning) compared to what SCG sources have been offering. But laser scanning functionality adds complexity and costs to the system and is typically limited to a range on the order of 100 nm. This is, in fact, on the lower end of what would be ideal for OCT.
Notably, these challenges could soon become a thing of the past.
Why SCG is challenging
Supercontinua are typically generated
by injecting an intense, ultrashort (femtosecond) laser light pulse into a transparent optical material. The spectral broadening of the pulse then essentially relies on
the intensity dependence of the transparent material’s refractive index. This is called the Kerr effect, and it results in the self-phase modulation of the injected pulse.
Put simply, the ultrashort pulse generates new frequency components through its own strongly varying intensity. The widest spectral bandwidths (at lowest input powers) are typically achieved in waveguide geometries, such as optical fibers or optical waveguides. This is because transverse confinement enhances pulse intensities to promote the generation of additional frequency components. Depending on the waveguide’s dispersion properties, SCG can be categorized into two broad domains (Figure 2).
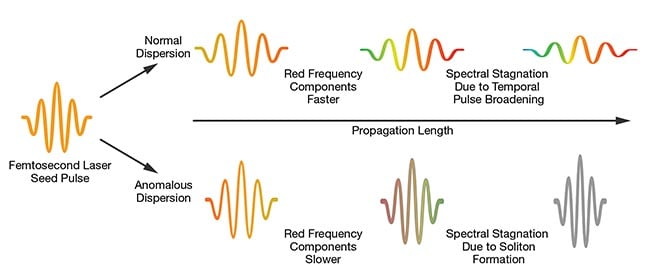
Figure 2. An illustration of supercontinuum generation in the normal dispersion regime (top) and the anomalous dispersion regime. Conventional supercontinuum generation (SCG) schemes suffer from spectral stagnation in either regime because the spectral broadening stops after a certain pulse propagation length in the dispersion medium. Courtesy of Menlo Systems.
In normal dispersion waveguides, where the dispersion coefficient is negative, the natural tendency is for “bluer” light frequencies to be delayed in the waveguide medium and for “redder” frequencies to travel comparatively faster. When combined with the addition of new frequencies in SCG, the effect accelerates
the dispersive broadening of the laser light pulse in time. Therefore, in normal
dispersion SCG, the pulse quickly loses
its peak intensity and any further band-
width generation ceases. Though the bandwidth is limited, the spectra produced are typically smooth. That is, there are few modulations across them.
Anomalous dispersion waveguides, in contrast, exhibit a positive dispersion
coefficient: The bluer frequencies are faster while the redder frequencies are slower, the exact opposite to normal
dispersion. In this case, the dispersion works to bring the newly generated frequencies together, such that the pulse temporally compresses, increases its peak power, and accelerates additional bandwidth generation. However, this only continues to a certain point.
Next, the pulse shapes itself into a stable configuration called a soliton, in which the nonlinear generation and dispersion work in opposition until no new spectral generation can take place. Anomalous-dispersion SCG usually generates larger bandwidths than normal-dispersion SCG, but the spectra are more modulated.
In either case, the effect of dispersion
causes spectral broadening to cease beyond a certain propagation length. This spectral stagnation eliminates the otherwise viable solution of increasing the propagation length to allow more interaction of the light with the material. In practical terms, one is then required to increase the optical input power by using a higher power laser. This is not only detrimental to waveguide-based SCG, as small cross sections imply limited damage thresholds, but also inefficient because the SCG bandwidth rises rather slowly with more optical input power. Obviously, these effects limit the efficiency and, therefore, the compactness of conventional SCG schemes. Typically, ultrashort laser pulses are employed, with energies in the 1 nJ/pulse range or above, which, in practice, translates into relatively costly, benchtop-sized devices.
Dispersion engineering is key
Whether in the regime of normal or anomalous material dispersion, conventional SCG suffers from spectral stagnation. The spectral broadening essentially stops after a certain pulse propagation length. To be more strictly precise, this limitation applies only as long as the medium’s dispersion characteristics are uniform along its interaction length.
What if, by employing both regimes of dispersion, the stagnation mechanism in either regime could be overcome?
Indeed, an answer to this question was recently proposed in the form of so-called patterned dispersion waveguides, which have now been developed to the level of perfection required for mass production and commercialization of on-chip SCG sources based on a silicon nitride platform1.
While dispersion patterning tends to yield a complex waveguide profile, in essence, the approach is to apply different dispersion regimes in a highly controlled fashion. The technology allows, on the one hand, the ability to overcome the loss of pulse peak power in normal dispersion due to stretching of the pulse in time, and, on the other hand, to prevent the anomalous dispersion’s stagnation of spectral bandwidth due to the pulse shaping into solitons.
The advantages are manifold. First, it allows the laser pulse bandwidth generation to be drastically increased. This decreases input power requirements down to factors on the order of 1/1000 as compared with other approaches, which implies a significant reduction in power consumption, size, and costs of suitable seed laser light sources (Figure 3). At the same time, the high-spectral-power ranges of the produced supercontinua can be massively broadened. Having the spectral power more balanced across the entire bandwidth is integral for high-
performance spectroscopic and interferometric applications such as OCT. Additionally, for nonlinear pulse compression applications, alternating dispersion
patterning can provide pulse durations <20 fs directly at the output.
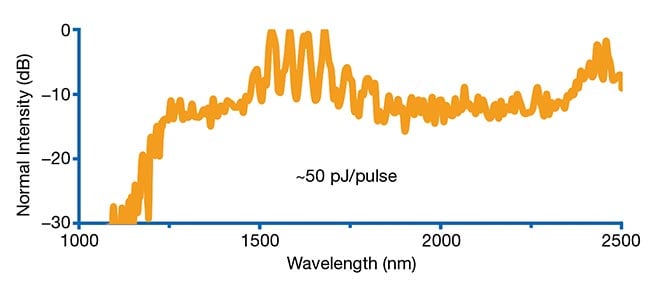
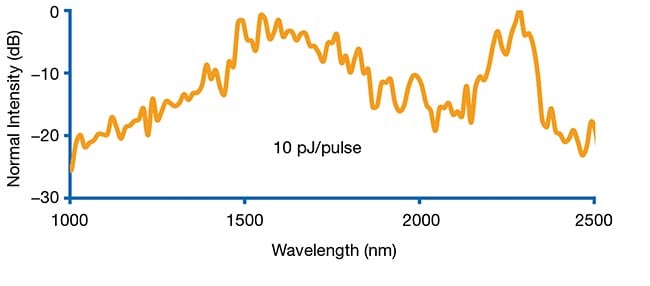
Figure 3. Examples of supercontinua generated in SuperLight’s patterned alternating dispersion on-chip waveguides employing Erbium-based femtosecond fiber laser oscillators. Over 1000 nm of bandwidth is generated by pulse energies in the range of ~50 pJ/pulse (top). Over 500 nm of bandwidth is generated by pulse energies as low as ~10 pJ/pulse (bottom). Courtesy of SuperLight Photonics.
Overall, alternating dispersion SCG is setting world records in the achievable ratio of bandwidth to input power. Pulse energies in the range of a few tens of picojoules are typically sufficient to generate >1000 nm of bandwidth. In extreme cases, the pulse energy required for wide-bandwidth SCG can be dropped to just a few picojoules. In practical terms, employing a femtosecond laser to deliver <100-fs pulses at a repetition rate of 100 MHz translates into ~1 mW of required average optical output power, which is lower than the average optical power emitted by a typical laser pointer.
Ultra-compact seed lasers
For conventional SCG, a considerable range of femtosecond laser designs are commercially available and readily meet common requirements with regard to the laser pulse energies and durations they deliver. Yet, when it comes to maximizing the compactness and cost-effectiveness of SCG systems, fiber-based femtosecond laser technology stands out as the technology of choice.
As to the portable laser market, battery-powered, wide-bandwidth supercontinuum sources are now better positioned to be implemented in crowded factory settings, field applications, or physicians’ offices.
Femtosecond fiber lasers can come engineered with modular designs incorporating mode-locked master oscillators to deliver pulses with tiny average output powers. This master oscillator output
can be subsequently amplified in one or several fiber amplifier stages. So far, these amplifier stages have been indispensable to support SCG with nanojoule pulse energies, at minimum, and suitable pulse durations on the order of 100 fs.
The ability of new patterned alternating dispersion waveguide schemes to reduce pulse energy requirements for SCG to a couple of picojoules changes the game, because it allows the option to employ unamplified oscillator modules on their own. This allows SCG possibilities to be completely rethought (Figure 4).
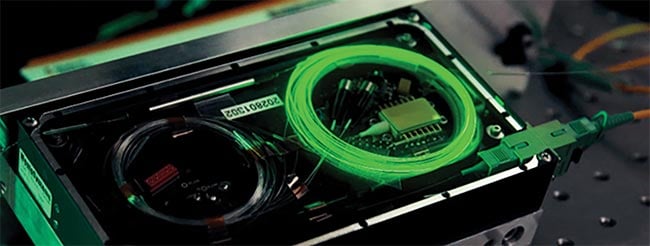
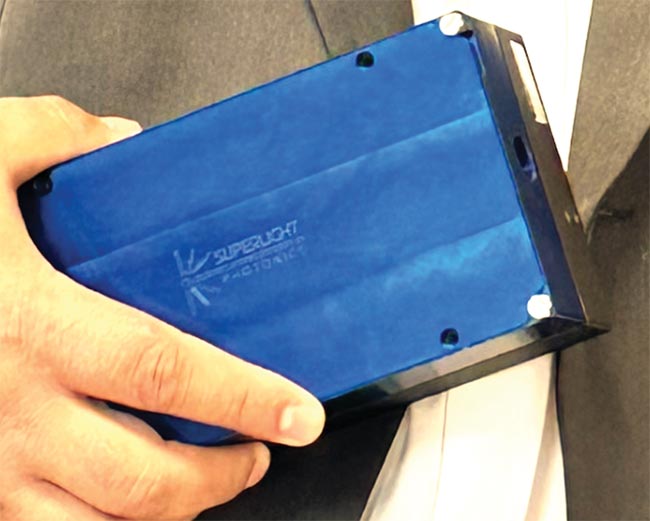
Figure 4. Supercontinuum sources leveraging novel dispersion patterned waveguide technologies enable reductions in pulse energy requirements down to a couple of picojoules (top). This changes the game for supercontinuum laser sources because it allows them to employ unamplified oscillator modules on their own. A figure-of-nine-based oscillator module readily fits in the palm of a hand and can be battery powered, which puts handheld supercontinuum lasers literally within reach (bottom). Courtesy of SuperLight Photonics.
This advancement goes hand-in-hand with state-of-the-art oscillator architectures for particularly robust femtosecond pulse generation, such as figure-of-nine oscillator schemes (named after the
characteristic shape of the laser cavity)2. The approach is based on an all-polarization-maintaining fiber design that efficiently decouples the laser cavity from perturbations, such as vibrations and
temperature variations, while implementing no critical or lifetime-limiting
components. Figure-of-nine-based oscillator modules readily fit in the palm of a hand and can be battery powered, which literally puts handheld supercontinuum lasers within reach.
The future at hand
Ultraefficient on-chip SCG, in conjunction with industry-proven femtosecond fiber laser designs, massively shrinks
the size and cost of accessible supercontinuum laser light sources. The technology overcomes the dispersion limitations of conventional approaches and is poised to advance laser-based sensing, ranging,
and imaging applications. As to the portable laser market, battery-powered, wide-bandwidth supercontinuum sources are now better positioned to be implemented in crowded factory settings, field applications, or physicians’ offices.
As dispersion-engineered SCG waveguides are implemented on the CMOS-compatible silicon nitride platform, it is tantalizing to envision mass-producible all-in-one chip architectures on the more distant horizon. A bright future of novel supercontinuum lasers seems to be at hand.
Meet the authors
Haider Zia is founder and CTO of SuperLight Photonics, a new startup aiming to advance ranging, sensing, and imaging through the
development of novel supercontinuum lasers. He holds a doctorate in nonlinear optical physics.
Jaroslaw Sperling is business developer
for femtosecond lasers at Menlo Systems, where he brings a passion for applied
photonics and years of experience in ultrafast laser spectroscopy. He holds a doctorate in physical chemistry from the University of Vienna.
Acknowledgments
The authors wish to acknowledge Christian Mauser of Menlo Systems and Cees Links of SuperLight Photonics for their assistance in preparing this article.
References
1. H. Zia et al. (2023). Ultraefficient On-Chip Supercontinuum Generation from Sign-Alternating-Dispersion Waveguides.
Adv Photonics Res, Vol. 4, No. 5.
2. W. Hänsel et al. (2017). All Polarization-Maintaining Fiber Laser Architecture for Robust Femtosecond Pulse Generation.
Appl Phys B, Vol 123, No. 41.