Nd:YAG Lasers: Standing the Test of Time
The Nd:YAG laser crystal was developed not long after the discovery of the ruby laser in the 1960s. Of all the solid-state laser crystals, it has stood the test of time best. Its high gain, narrow linewidth, low threshold and physical properties make it a most versatile laser material for a variety of applications.
Quantel USA
The ubiquitous Nd:YAG laser has played many roles over the years. For the military, it has provided rangefinding and target designation capabilities. When used with nonlinear optics or as a pump source for other lasers, it offers scientific users diversity in pulse width format and lasing wavelength. Other applications include medicine (dermatology and ophthalmology), materials processing (cutting, trimming, welding and surface cleaning) and commercial instrumentation (ablation, spectroscopy, marking, nondestructive testing and light shows). Nd:YAG lasers and their harmonic version are used under harsh environmental conditions for remote sensing, gated imaging illumination, bathymetry, ocean and atmospheric studies, and many other real-world applications that require compact, rugged sources.
In some of these application areas, Nd:YAGs compete against nonphotonic techniques and other lasers, such as CO2 and high-power diode lasers. But the Nd:YAG offers a wider application range, in large part because of its unique properties and because it is one of the few lasers that operates efficiently with either flashlamp or diode pump and in pulsed or continuous-wave (CW) mode.
The crystal
Nd:YAG is a man-made cubic garnet crystal (Nd:Y3Al5O12) grown by the Czochralski method. A high-purity oxide powder compound of aluminum, yttrium and neodymium (the active dopant ion that substitutes into yttrium sites with a doping level of about 1 percent) is first placed in an iridium crucible and melted in a radio frequency furnace at about 1970 °C. A seed crystal is then brought into contact with the liquid surface. When slowly lifted, rotated and cooled slightly, a high-quality, single-crystal boule of neodymium-doped yttrium aluminum garnet (Nd:YAG) emerges at the rate of about 0.5 mm per hour.
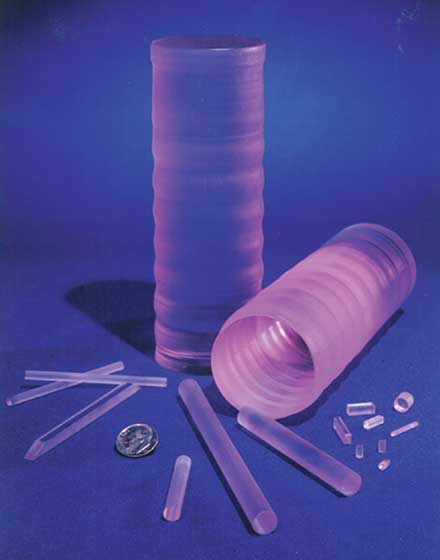
Figure 1. Boule with rods extracted from it.
Typical crystal boules are 60 to 80 mm in diameter by 175 to 225 mm in length. Rods, wafers and slabs in various geometries are extracted from the boule and then fabricated, polished and coated to customer specifications (Figure 1). Finished products range from diode-pumped laser rods as small as 0.5 mm in diameter by 25 mm long to slab geometries as large as 8 × 37 mm in cross section by 235 mm long. More than two-thirds of Czochralski growth station volume in the US is in Nd:YAG, making it the most widely used laser crystal. Other YAG crystals being grown in volume are Er:YAG and CTH:YAG.
The resonator
The most common Nd:YAG rod geometry is a right circular cylinder, although slabs, wafers and rectangular shapes sometimes are used. The rod end faces usually are polished flat and parallel (or oriented near Brewster’s angle for low transmission loss without optical coatings). Convex radiused rod faces sometimes are used to compensate for thermal lensing. In its simplest form, the resonator comprises the rod with a highly reflective dielectric coating at one end and a partial reflector (outcoupler) at the other. The end faces must be precisely parallel in this case. The more typical configuration uses separate mirrors at each end of the resonator along with other optics and crystals added to polarize, modulate, Q-switch and redirect the beam, select net lens power, convert wavelength and spectrally separate its output or a combination of these effects.
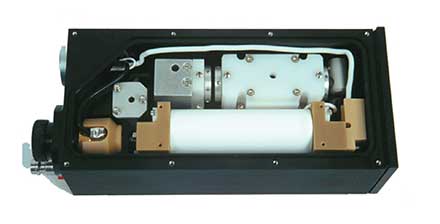
Figure 2. Resonator layout in the Ultra CFR.
The resonator can be stable or unstable. Most scientific lasers use graded-reflectivity mirrors to produce low beam divergence, moderately high energy and therefore high brightness. Many commercial applications do not require a tightly focused spot, and the more conventional multimode stable resonator often is used. The lowest order stable transverse mode, TEM00, has a smooth, Gaussian spatial beam profile in both the near and far field. Most stable resonators are operated multimode; power (or energy if pulsed) is much higher because the lasing diameter can be larger than if confined to the single TEM00 mode. The application dictates the choice of single- vs. multimode operation.
CW Nd:YAG lasers of more than 5 kW and flashlamp-pumped lasers of more than 20 J have been built. Almost any pulse width from ultrashort to CW is possible. Electro-optically Q-switched, pulse-pumped Nd:YAGs typically produce 5- to 20-ns pulse widths. Lower gain, acousto-optically modulated CW-pumped Nd:YAGs produce 150- to 300-ns pulses. Relatively simple oscillator-amplifier configurations can produce 1 to 2 J of Q-switched energy at 1064 nm.
TABLE 1.
Nd:YAG PROPERTIES AND THEIR BENEFITS
|
Nd:YAG Property |
|
Value
|
|
Major Benefit |
|
Lasing wavelength |
|
1064 nm |
|
Convertness; silicon detector response for receivers; visible harmonic wavelengths available at green and blue; NCPM OPO at eye-safe wavelength |
|
|
|
|
|
|
|
Linewidth |
|
4.5 Å |
|
Ease in phase matching spectrally; good optical pumping efficiency |
|
|
|
|
|
|
|
Fluorescence lifetime |
|
230 µs |
|
Convenient for flashlamp or diode pumping |
|
|
|
|
|
|
|
Stimulated emission
cross section |
|
3 x 10—19 cm2 |
|
High gain, narrow pulse width, low
saturation fluence and low insertion
loss of laser optics
|
|
|
|
|
|
|
|
Thermal conductivity
(at 300 K) |
|
0.14 W/cm—K |
|
Tolerable thermal lensing/birefringence loss |
|
|
|
|
|
|
|
Hardness |
|
8 — 8.5 Mohs |
|
Ease of rod fabrication without breakage; good optical quality and robust material |
|
|
|
|
|
|
|
Index of refraction |
|
1.8 |
|
Convenient for AR coating with MgF2 |
Limitations arise because of the high gain (parasitic effects) and safe operating fluence. Active mode-locking schemes allow pulse widths from about a fraction of a nanosecond down to a few picoseconds. Shorter pulse durations require lasing media with higher gain bandwidth such as Ti:sapphire. Non-Q-switched long-pulse lasers also can produce pulse widths of 100 ms or more. This diversity in pulse duration makes it possible to deliver a wide range of peak power density to suit specific applications.
The diode-pumped Nd:YAG laser has opened up a wider range of applications, thanks to its increased source stability, efficiency and lifetime, and reduced power consumption and size. Commercial Nd:YAG lasers with repetition rates higher than 100 kHz are now available. Diode-pumped devices are best suited for low-pulse-energy or CW power applications because the price of diode bars/arrays still is much higher than that of linear flashlamps.
Frequency-doubled to the green at 532 nm, tripled to the blue at 355 nm and quadrupled to the deep blue at 266 nm are other key Nd:YAG wavelengths. The 532 nm wavelength is ideal for pumping Ti:sapphire or dye lasers (in liquid or plastic matrix form). Noncritically phase-matched KTP optical parametric oscillators (OPOs), when pumped with a Q-switched Nd:YAG at 1064 nm, efficiently generate 1.57-µm radiation, a more eye-safe wavelength for use in less controlled areas. The laser also has lower gain wavelengths near 1.32 and 1.44 µm and 946 nm that can be frequency-doubled, frequency-mixed or Raman-shifted to provide visible and near-IR wavelengths for specialty applications. The diversity in wavelengths when coupled to nonlinear optics and OPOs is unique.
Linewidth
Linewidth is a measure of spectral purity (monochromaticity). For the cubic structure of Nd:YAG, linewidth is about 30 to 50 times narrower than Nd:glass, for example, making Nd:YAG relatively easy to phase match spectrally with harmonic generators to visible wavelengths. On the downside, the absorption spectral linewidth for pumping is quite narrow, making diode pumping somewhat more demanding than it is with Nd:YLF, for example.
The fluorescence lifetime is a measure of how quickly the metastable state depopulates after optical pumping. The fluorescence lifetime of Nd:YAG is about 230 µs, nearly ideal. If much shorter, as with Ti:sapphire or dye lasers, the stored energy would radiate away spontaneously unless extracted quickly from the resonator. Thus, flashlamp pumping is less efficient, and high peak lamp current densities are required. If much longer, as in Er:YAG, it is more difficult to extract the stored energy efficiently in a short pulse because the gain is lower.
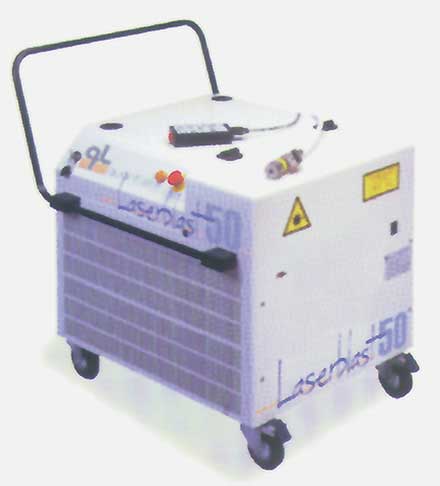
Figure 3. Laserblast Cleaning System. Courtesy of Quantel SA.
For pulsed operation, a flashlamp current pulse duration of 150 to 200 µs often is used because the stored energy doesn’t radiate away, and sufficient input pump energy can be delivered in that time to produce practical output energy levels with long flashlamp life. The Nd:YAG can be CW or pulse-pumped with lamps or diodes. Diode pumping near 808 nm substantially increases efficiency compared with broadband gray body pump sources using xenon- or krypton-filled flashlamps.
The stimulated emission cross section is a measure of lasing efficiency and gain. For Nd:YAG it is a very favorable 3 × 10–19 cm2. This high gain is well-suited for short Q-switched pulses and low pump threshold energy. If the figure were higher, amplified spontaneous emission and parasitic effects would further limit the practical maximum stored energy density in the rod. The saturation fluence for Nd:YAG of around 500 mJ/cm2 is well-suited for efficient energy extraction from amplifiers at safe fluence levels.
When the stimulated emission cross section is lower, as in Nd:glass, the gain is much less, the insertion loss of optical components in the resonator is higher and the Q-switched pulse width is wider. Nd:glass, with its lower cross section and wider linewidth, is better suited for higher energy-storage density and shorter mode-locked pulse width.
Thermal properties
The combination of thermal expansion coefficient, conductivity and index of refraction change with temperature (dn/dT) in Nd:YAG provides acceptable thermal lensing and birefringence loss except for large rod diameters and moderate pulse repetition rate. Nd:glass has one-fifteenth the thermal conductivity of Nd:YAG, so it is much more limited in repetition rate and maximum thermal loading limit to fracture. Nd:YLF has thermal lensing and natural birefringence characteristics that are superior to Nd:YAG. Table 2 summarizes the differences between Nd:YAG and laser materials whose fundamental wavelength is similar.
TABLE 2.
Nd:YAG COMPARED WITH Nd:YLF/Nd:GLASS
|
Property |
|
Nd:YAG |
|
Nd:YLF |
|
Q-88 Nd:glass
|
|
Lasing wavelength
|
|
1064 nm
|
|
1053 nm
|
|
1054 nm
|
|
|
|
|
|
|
|
|
|
Linewidth |
|
4 — 5 Å
|
|
9 — 10 Å |
|
220 Å |
|
|
|
|
|
|
|
|
|
Fluorescence lifetime
|
|
230 µs |
|
480 µs |
|
330 µs |
|
|
|
|
|
|
|
|
|
Stimulated emission cross section
|
|
3 x 10—19 cm2 |
|
1.5 x 10—19 cm2 |
|
4x 10—20 cm2 |
|
|
|
|
|
|
|
|
|
Thermal conductivity
|
|
0.14 W/cm-K
|
|
0.06 W/cm-K |
|
0.01 W/cm-K |
|
|
|
|
|
|
|
|
|
Hardness |
|
1215 (Knoop)
|
|
4 — 5 Mohs
|
|
418 (Knoop)
|
|
|
|
|
|
|
|
|
|
Index of refraction
|
|
1.82 |
|
1.46 |
|
1.55 |
Nd:YAG has the hardness of mild steel. It is a very robust material that allows polishing to a high-quality finish and, unlike Nd:YLF, is neither fragile nor soluble in water. Its index of refraction of 1.8 makes it particularly easy to apply an antireflection coating at 1064 nm with a single layer of MgF2 for low transmission loss. Multilayer coatings are used on Nd:YLF and Nd:glass because their indices of refraction are lower.
It’s easy to understand why Nd:YAG is the workhorse solid-state laser material. It has near-optimum mechanical, optical and lasing media properties for diverse applications. In lasers, it can deliver a very wide range of pulse width, energy per pulse and repetition rate. When used with nonlinear optics, parametric oscillators or other lasing media as a pump source, wavelengths from deep blue to mid-IR are available. It can be efficiently diode or flashlamp pumped and used easily in pulsed or CW mode. No other solid-state laser material can claim all these attributes. Ubiquitous is a fitting description.
/Buyers_Guide/Keopsys_by_Lumibird/c1758