Advancements in automated motion control are helping high-volume manufacturing processes meet the demands of the consumer electronics and semiconductor industries.
MARIE FREEBODY, CONTRIBUTING EDITOR
Laser micromachining has proved to be revolutionary in modern manufacturing,
enabling the creation of intricate features at the micro- and nanoscale. Laser micromachining and microprocessing envelop various similar, yet distinct, processes. These include laser patterning and texturing, engraving, scribing, and cutting. Engineers in a range of industries have relied on these and other similar processes for decades.
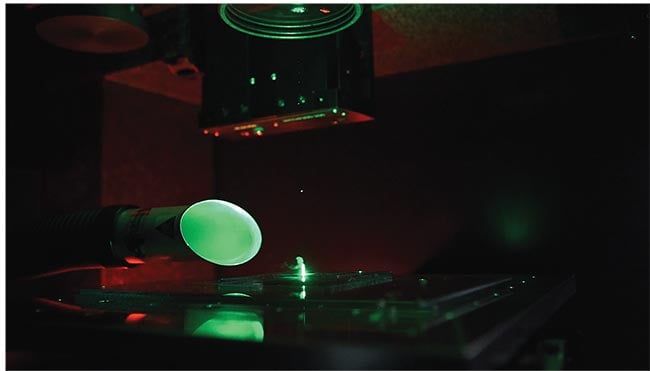
Courtesy of Fluence.
Today, the semiconductor and consumer electronics industries provide the powerful tailwinds driving progress in this technology area. Advanced manufacturing can be qualified by the high-tech innovations that the industries that power the market are delivering. It is also characterized by the sophistication of the processes and components that enable their manufacture.
In either case, laser micromachining and microprocessing are not the only technologies behind this trend: Another, motion control, is central to the success of laser-based manufacturing. Motion control is essential for advanced manufacturing applications, facilitating the degrees of system precision and efficiency that are necessary for high-volume production as well as the tight part tolerances that are required in precise machining.
If there is a hierarchy for motion control, commoditized capabilities exist at the lower end as typically inexpensive and ubiquitous solutions. At the higher end, a push for automation to reach new levels of precision is driving growth and development of new product solutions.
“Market development in electronics
and semiconductor environments is expected to continue to move fast,” said Amanda Dobbins, director of business development at SCANLAB GmbH, a developer of laser scan systems used in a range of laser-based manufacturing applications. “Continuous evolution on the product side will require more precision and throughput in manufacturing and processing technologies. Also, the demand for advanced scanning solutions will push R&D for laser systems.”
As demand increases for higher throughput and tighter part tolerances, the approach to controlling laser positioning systems has become more stringent. This is evident in applications that require high-speed methods, such as laser scan heads that are used to control beam position.
“For the customers I work with, accuracy is typically the number one driver when it comes to considerations for a micromachining system,” said Matthew Tedford, photonics central applications manager of precision medicine and manufacturing at Novanta. “From my experience, the demand has been typically for 10 µm (±3σ) or better for a system-level accuracy spec. More recently, I’ve seen applications start to push this to about 5 µm (±3σ) or better.”
Priority considerations
According to Jeff Hansen, senior principal applications engineer at MKS/Newport, the evolution of motion control technology has reached a point at which the technological capabilities necessary for many widespread applications are now available to a broad customer base. The technology itself has undergone rapid advancement. This, Hansen said, is crucial to remain competitive as well as to meet the continuously growing demands of industry.
“Initially, the cost of implementing motion control systems was a major concern,” Hansen said. “Over time, as technology has advanced and become more accessible, the cost factor has become more manageable, allowing for broader adoption.”
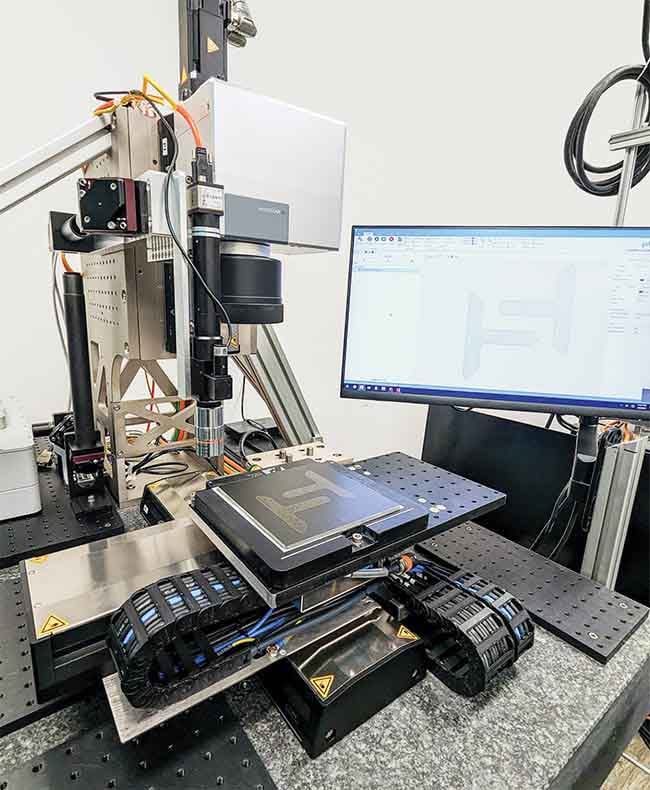
SCANLAB partners with Turner Laser Systems for application development and scan system integration. A challenge involves the integration of real-time control capability with the interface to effectively combine the movements of a stage and the scan system.
Courtesy of SCANLAB.
In the past, stage manufacturers provided not only the control for the stage but also the control for the galvanometer — typically a scan head. Although pairing the galvanometer with the stage increases throughput, the approach also increases system costs.
Industry has taken steps to address the dynamic, with the aim to streamline the motion control aspect of a system to ultimately enable improved accuracy and features through the laser micromachining process. In 2007, precision motion and automation firm Aerotech unveiled a coordinated stages and galvanometer solution. The company now markets a line of controller products that alleviates challenges posed by non-coordinated control elements.
Innovation has continued. Earlier this year, SCANLAB, in partnership with ACS Motion Control, announced a solution that combines a 2D scan head with an xy positioning stage in which the scanner and stages are continuously moving during operation.
Novanta has also developed and introduced an approach — one that places priority on reducing the hardware and software needed to enable a coordinated system to become operational.
The focus on this consideration aligns with what manufacturers are coveting: streamlined solutions delivering high throughputs.
“Manufacturers ideally would like to have ‘lights out’ factories, and the only way to get to this is through motion control and automation,” Tedford said. “I expect this will lead to different and potentially novel ways to use existing technologies.”
Communication in motion control
systems, especially for today’s systems that rely on coordinated elements, is necessary to sustain any shift toward automation on the road to the streamlined work environments that manufacturers seek. In motion control systems, control mechanisms and the physical terminals (endpoints) of a given operation must
be able to send and obtain information. The communication function between
the two elements is enabled by bus protocols.
On the design side, motor designs are also frequent areas of both novel solutions and iterative product improvements. These include direct-drive linear motors as well as piezoelectric actuators. Direct-drive linear motors eliminate the need for mechanical transmission elements, which reduces backlash and increases responsiveness. Piezoelectric actuators meanwhile are known for their high precision and responsiveness and are particularly valuable in applications that require submicron levels of accuracy.
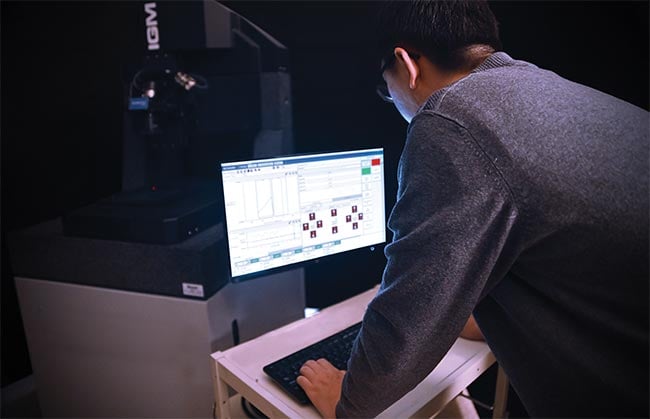
Powerful embedded controller devices with lower latency bus protocols lead to higher trajectory rates, which also influences precision for laser scan head applications, according to Bryan Germann, product manager for laser processing products at Aerotech. Courtesy of Aerotech.
To ensure lasers can be positioned quickly and accurately to meet the demands of modern manufacturing processes, advancements in drive hardware also play a pivotal role.
“Advancements in more powerful embedded controller devices with lower latency bus protocols are enabling higher trajectory rates, which also impacts
precision for laser scan head applications,” said Bryan Germann, product manager for laser processing products at Aerotech.
The ascent of automation
The advantages to motion control automation are multifaceted: The incorporation of an automation element can significantly enhance both quality and cost-
efficiency in manufacturing. This is proved by significant cost savings in areas such as labor, material waste, and energy consumption.
Functions and applications that benefit from motion control automation include semiconductor wafer processing, battery processing, and solar panel processing. “Equipment manufacturers selecting precision motion control equipment require specialized tools to achieve their application objectives,” Germann said. “Superior electromechanical design and controller
features that enable synchronizing the laser and part motion are extremely
enabling for difficult laser applications.”
Automation also offers preventative benefits, helping manufacturers avoid human and operational errors that could lead to lower yields.
“Manufacturers ideally would like to have ‘lights out’ factories, and the only way to get to this is through motion control and automation.”
Automation can also bring advanced functionalities to micromachining workstations. Features such as real-time monitoring, automatic adjustments, and predictive maintenance become possible, leading to more robust and reliable systems.
“The ability to quickly and precisely modify laser processes in-line with the help of vision systems, sensors, and other peripheral equipment ensures accuracy throughout the process, and thus higher yields,” Dobbins said.
Again, the incorporation of automation ties to the relationship between the operating laser and the scanner.
“The integration of process monitoring to assure highest product quality requires tight synchronization between laser and scanner,” Dobbins said. For printed circuit board (PCB) manufacturing, for example, xy tables are commonly used. For drilling the connections between layers of a board, the PCB substrate is positioned beneath a laser scan head. Copper, which is highly reflective in the CO2 wavelengths (~9.3 to 10.6 µm), can be opened on the PCB using an ultraviolet laser. The PCB is then positioned under a CO2 laser system to drill through the epoxy laminate material.
“Because the area the scan head can process is smaller than the PCB board, the positioning of the substrate under the scan head is critical,” Tedford said.
“And because you don’t have the two laser wavelengths going through the same scan head, the motion control stage and machine vision plays a critical role in making sure the substrates are positioned correctly for drilling.”
Motion control and software interplay
A motion controller interfaces with physical motion hardware, such as motors, stages, drives, and laser scan heads. To operate effectively, motion controllers require both firmware and software. Firmware, embedded in digital signal processors or field-programmable gate arrays, generates the current signals for servo motors and interfaces with feedback devices, such as encoders, for accurate positioning.
In certain cases, such as very high-speed automation, the motion controller is treated as an independent control system. To ameliorate the burden on the motion controller, automation is carried out on a software layer between the main automated system and the software that drives the galvanometer controller.
“Communication between the [galvanometer] controller and the main programmable logic controller (PLC) is critical for high-speed execution,” said Mark Turner, founder and CEO of Turner Laser Systems. “However, in some cases, the amount of control required is too complex and requires the controls algorithms to be done on a software level, not on a motion controller level.”
Obtaining the measurements of the position, scale, and skew errors of a part, and then transforming the part in software before uploading it to the motion controller, is one such example, according to Turner.
A challenge is to integrate real-time control with the interface, to combine the movements of a stage and the laser scan system.
“We realized that micromachining today requires more and more precision regarding physical movements and
motion control, together with managing the laser power efficiently,” Dobbins said. The company uses software to calculate optimum trajectories from specified
machining patterns and process parameters, she said. The calculation considers the physical limits of the scan head.
“This allows us to achieve optimal processing results with minimum laser-off times,” Dobbins said.
The rise of ultrafast lasers
In modern manufacturing, high-peak-power ultrafast lasers have allowed laser micromachining processes to reach new standards for the quality and precision of micro- and nanoscale features. However, the proliferation of these lasers has not bypassed bottlenecks, especially as average powers have increased. Ultrafast lasers, capable of generating multiple wavelengths from a single source, present challenges for optical damage thresholds and supported wavelengths for laser scan heads. This necessitates high-quality optical coatings with high laser-induced damage thresholds (LIDTs) in multiaxial laser scan head products.
According to Turner, a 50- to 100-W ultrafast laser, which needs to be moving very fast or use beamsplitting to reap the benefits from the source, presents a good example.
“Power scaling can often affect the commercial viability as it drives throughput, and thus cost-per-part. So, the motion control is critical for high throughput ultrafast applications,” Turner said.
Moreover, flexibility in pulse output methods, such as generating megahertz or even gigahertz repetition rates with burst mode, indicates the importance of high-quality laser-triggering electronics.
“Accurately triggering these pulses with respect to micrometer or submicrometer positioning systems highlights the importance of high-quality laser triggering electronics for scanners,” Germann said. “Everything operates faster and on smaller time scales, so there is increased design pressure on control electronics and methods.”
Today’s control systems achieve precision and accuracy using high-resolution encoders and advanced control algorithms. These systems also typically incorporate real-time compensation mechanisms for various disturbances, which further ensures stable and precise operations.
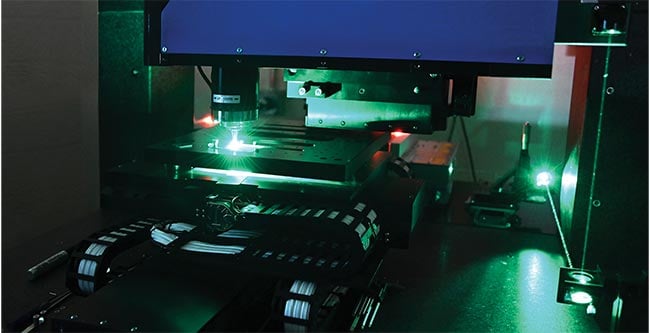
Aerotech’s 515-nm, five-axis laser micromachining center with drilling operation using a femtosecond laser. Courtesy of Aerotech.
Increasingly, designers are integrating other types of advanced technologies, such as AI and machine learning algorithms, that aim to dynamically optimize the laser processing parameters. These technologies allow for adaptive control, meaning that the system can adjust in real time to changes in environmental conditions or material properties.
Changes to material properties, particularly amid the wide adoption of ultrafast laser technology, underscores the persistent — and critical — need to optimize the light-matter or light-material interaction.
Position synchronized output (PSO), a function of a control mechanism that
coordinates motion with output to the laser, is a favored solution to control this interaction. Aerotech’s PSO solution, for example, offers users several operating modes that accommodate the need for precision integration with a range of distinct processes.
“Control of the laser-material interaction is crucial, and PSO is an enabling technology for both motion control and ultrafast laser processing,” Germann said.
As demand increases for higher throughput and tighter part tolerances, the approach to controlling laser positioning systems has become more stringent.
“Functions such as PSO pushed the development of ultrafast lasers toward minimizing the jitter between the triggering signal and the actual pulse coming out of the laser head to increase the processing quality and accuracy,” said Bogusz Stepak, director of Fluence’s Ultrafast Laser Application Laboratory.
“As a result, the processing time can be shorter, and the sharp corners can be machined with better quality.”
Choosing the right platform
Most commercial motion control solutions support the full range of laser micromachining tasks. Physics dictates many considerations pertaining to system setup, including laser wavelengths, beam diameters, and optical configurations needed to achieve the desired spot size, Rayleigh length, and pulse energy.
Still, the optimal solution must address specific application needs, which may include payload, travel, speed, precision, and cost.
“End users can drive their applications to success by having the right skill sets and understanding the capabilities of the tools at their disposal that dictate success,” Germann said. “Every application involves a laser and a motion system, [and it is] application requirements, such as feature size and material type, that end up dictating which tools are most appropriate to do the job.”
Applications that demand large spot sizes and slower processes, such as welding, do not exclusively necessitate ultrafast lasers. Users can commonly manage these functions with the use of lower dynamic scanners and stages or robots with average accuracy requirements. Conversely, applications involving high-peak-power ultrafast lasers necessitate high LIDT mirrors, high dynamic motors, and complex motion with higher accuracy requirements.
At Fluence’s application laboratory,
feasibility studies determine the best source and required optics for a given
material and process. According to Stepak, these studies can also determine
the most efficient delivery of laser
energy as well as the scanning strategy to maximize the average laser power while maintaining processing quality.
“In the ultrashort-pulse domain, process optimization is a complex task due to multidimensional parameter space and the high sensitivity of the process performance to the laser parameters,” Stepak said. “This will determine whether the beam motion should be implemented by combining the [galvanometer scanner] and linear stages or fixed high-numerical aperture optics.”
In some cases, it is beneficial to combine the scanner with a microscope objective for tight laser focusing. Modern lasers often offer more power than that which can be used with a single beam, which means that the main beam can be split into several or multiple spots using beam shapers.
“Femtosecond lasers often provide the highest processing quality and precision due to reduced thermal effects and a
melt-free process,” Stepak said. Today’s class of industrial-grade femtosecond
lasers meet the demand of mass production, after available powers increased significantly during the last few years,
he said.