Photonics HandbookTechnology News
Micro-rings Make Green Gap Wavelengths Accessible for Tiny Lasers
Compact, high-quality lasers that generate red and blue light have been available for years, but lasers that emit light at yellow and green wavelengths and are small enough to fit on a chip have been harder to build. Wavelengths between 532 nm and 633 nm, commonly referred to as the “green gap,” are especially challenging to produce with conventional laser gain. Green laser pointers produce light only in a narrow spectrum of green, and because they are not integrated in chips, they cannot work with other devices.
The ability to develop stable, miniature lasers in the yellow and green regions of the visible light spectrum — the infamous green gap — could open new opportunities in many areas, from undersea communications and quantum computing to medical treatments and displays.
At the National Institute of Standards and Technology (NIST), scientists have found a way to close the green gap by using a ring-shaped microresonator small enough to fit on a chip. By changing the dimensions of the microresonator, the researchers extended its range to wavelengths as short as 532 nm.
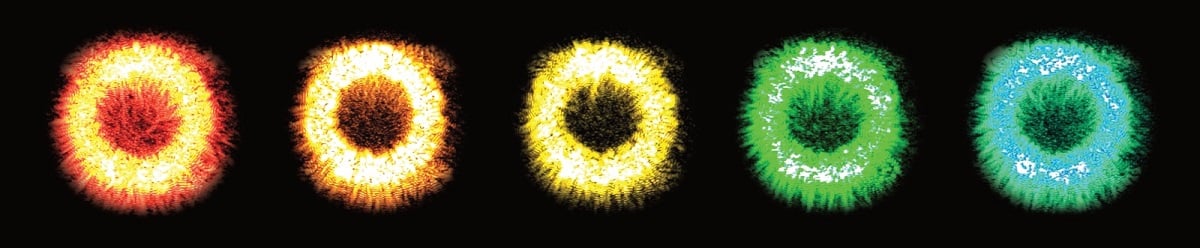
A series of visible light colors generated by a microring resonator developed by NIST researchers. Courtesy of S. Kelley/NIST.
Their solution is based on earlier work by NIST Fellow Kartik Srinivasan and his team. In previous studies, the researchers used microresonators composed of silicon nitride (Si3N4) to convert IR laser light into other colors.
They pumped IR light into a ring-shaped resonator, where it circled thousands of times until it reached intensities high enough to interact strongly with the Si3N4. The interaction created an optical parametric oscillation (OPO) that produced two new wavelengths of light, called the signal and the idler. The signal had a wavelength in the visible range. The idler had an IR wavelength that was longer than that of the pump laser.
The researchers demonstrated that a few individual colors of visible laser light in the red, orange, and yellow wavelengths could be generated by altering the dimensions of the microresonator. However, they could not generate the full complement of yellow and green colors necessary to fill the green gap.
“We didn’t want to be good at hitting just a couple of wavelengths,” researcher Yi Sun said. “We wanted to access the entire range of wavelengths in the gap.”
The researchers proceeded to increase the thickness of the microresonator, optimizing the microring geometries for green gap emission and enabling the light generated by the resonator to penetrate deeper into the green gap.
Using a dispersion engineering technique, they exposed the microresonator to more air by etching away some of the silicon dioxide (SiO2) layer underneath it to create an undercut. This step made the output colors less sensitive to the microring dimensions and the IR pump wavelength, giving the researchers more control over the slightly different green, yellow, orange, and red wavelengths they could generate from their devices.
The researchers accessed the entire green gap with the OPO in the Si3N4 microrings pumped near 780 nm.
Using just four devices, they were able create more than 150 distinct wavelengths evenly distributed across the green gap, as predicted by dispersion simulations. They further established the usefulness of OPO to coherent applications by demonstrating continuous frequency tuning of greater than 50 GHz and narrow optical linewidths of less than 1 MHz.
“Previously, we could make big changes — red to orange to yellow to green — in the laser colors we could generate with OPO, but it was hard to make small adjustments within each of those color bands,” Srinivasan said.
Now that it has closed the green gap, the team is investigating how to produce the green gap laser colors more efficiently. Currently, the output power is only a few percent of that of the input laser. Improving the coupling between the input laser and the waveguide that channels the light into the microresonator, along with developing better methods of extracting the generated light, could improve the efficiency significantly.
A miniature source of green laser light could be useful in underwater communications because water is nearly transparent to blue-green wavelengths in most aquatic environments. Other potential applications for wavelengths in the green gap include full-color laser projection displays and laser treatments for diabetic retinopathy and other medical conditions.
Compact lasers that encompass the green gap wavelength range could potentially be used in quantum computing and communications to store data in qubits. Quantum applications currently depend on bulky, powerful lasers that limit their use outside of the laboratory. Access to the green gap wavelengths could also help bring nonlinear nanophotonics and its many advantages to the visible spectrum.
The research was published in Light: Science & Applications (www.doi.org/10.1038/s41377-024-01534-x).
Published: September 2024