BRYAN GERMANN, AEROTECH
Additive manufacturing, also known as 3D printing, has revolutionized the manufacturing industry by enabling the creation of complex and customized parts with unprecedented precision and flexibility. Among the various additive manufacturing techniques, two-photon polymerization (2PP) has emerged as a powerful tool for creating high-resolution structures at the nanoscale. The impressive resolution of this technique presents unique scalability challenges due to the submicron laser spot sizes required to enable minimum voxel size (routinely <150 nm3). Scalability is challenged further by the limited field of view (FOV) of the laser scanner.
These process-specific attributes of the 2PP process can cause extremely long printing times and part printing defects due to FOV stitching.
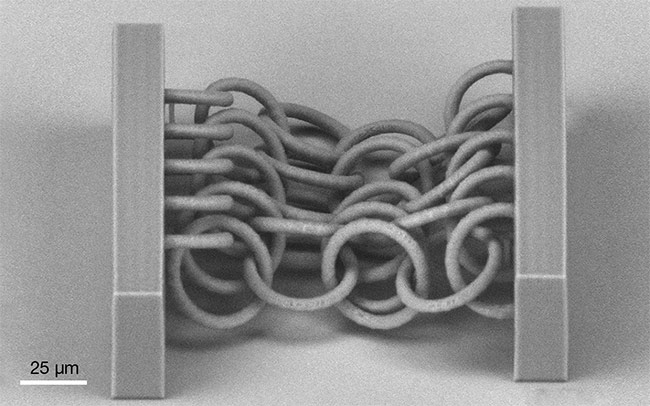
Standard 3D printing struggles to produce functionally intertwined structures, such as chain mail, because these structures must be supported physically during production. Multiphoton polymerization techniques, in contrast, can print structures suspended inside a gel or liquid monomer, which allows for support-free 3D printing. Courtesy of Femtika.
In recent years, advancements in motion control technology have offered alternative solutions for overcoming these challenges and enabling 2PP system builders to significantly improve production efficiency.
Before delving deeper into the engineering that underlies this motion control solution, it is essential to understand
the basics of multiphoton polymerization and its relevance to additive manufacturing.
Like its lower-resolution sister technique, stereo-lithography, which leverages a laser positioned in a planar layer to harden a photosensitive polymer liquid, 2PP is a technique that uses ultrashort laser pulses from a femtosecond laser to induce multiphoton polymerization. The laser pulses from a femtosecond source are so short that targeted polymer chains cure only at the laser’s maximum focal point. This means that a volume of polymer can be hardened in free space and with such low mass that it holds its position in the surrounding liquid. Repeating this process at select X/Y/Z positions within the liquid volume enables a layer-by-layer creation of a desired solid structure. The high-precision, fine resolution, and free-space curing of polymer chains in a zero-support framework make 2PP processes ideal for microfabrication, micro-optics, and biomedical engineering applications.
The laser spot in a 2PP system is
routinely positioned using a laser scan head, which moves the focused beam over an area by moving reflective mirrors through a focusing objective. One of
the key challenges, however, is that
the limited FOV of the laser scan head determines the maximum size of the structure that can be printed. High
numerical aperture objectives, which
are necessary for minimizing the spot
size of the laser, compound the challenge by further reducing the working distance and FOV. As a result, printing large
structures or multiple structures
in a single scan is difficult without using linear stages to move the scanner or the printing bed.
Beyond the limits of scanner field
The FOV constraints associated with laser scan head optics, which produce a small spot size, are not restricted to the submicron process needs of 2PP. All laser processes that leverage a laser scan head for beam motion routinely run into these constraints. Such complications have prompted the development of advanced new controller features that allow the extension of the FOV.
The most common method for achieving this behavior is FOV stitching, in which the motion within one scanner’s FOV is executed before the linear servo stages move the FOV over one full width. This allows the next FOV to be processed. This process is repeated until the entire desired area is processed.
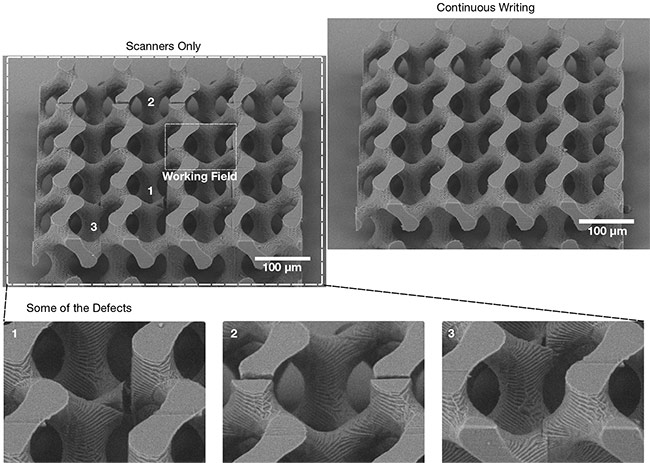
Examples of the inconsistent stitching errors commonly associated with using a step-and-repeat stitching method for extending the laser scan head field of view (FOV). With infinite field of view (IFOV), (top right) note the elimination of the boundary errors in the same feature. Courtesy of Femtika.
This method generates opportunities for more advanced control approaches that help eliminate boundary errors from the stitch process — for example, a feature that requires multiple stitched FOVs may result in gaps or discontinuities in the processed material. In the 2PP process, such errors result in stress points, missing features, or over- or mis-cured areas of the print.
A more effective way to achieve precise trajectories that extend beyond a lens’s FOV limit is to move both the laser beam and the processed component in a simultaneous fashion. This enables an infinite field of view (IFOV).
IFOV allows for the small spot size and FOV of the high-numerical aperture lenses required to apply a 2PP process over the entire linear stage area without any loss of positioning speed or resolution.
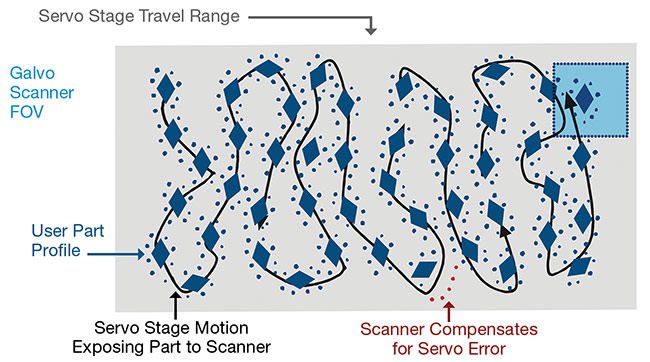
A representation of how infinite field of view (IFOV) leverages linear XY servo axes to extend the field of view (FOV) of a laser scan head. As the scanner FOV moves along the servo stage motion, it can process any features within the FOV at that instant. Courtesy of Femtika.
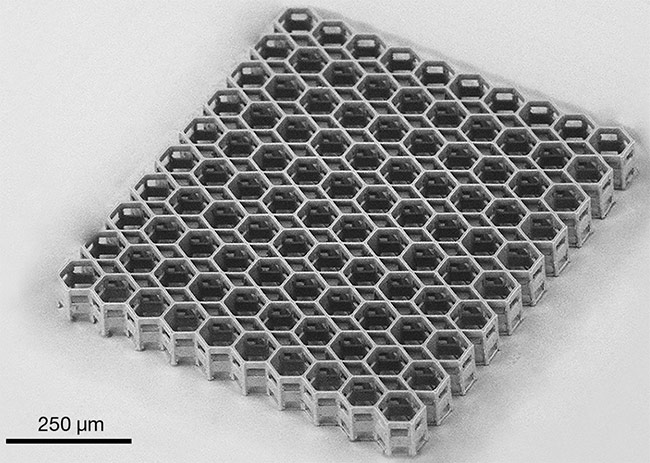
Multiphoton polymerization techniques with a resolution of up to 150 nm can produce scaffold-like structures with submicron-sized cells. Courtesy of Femtika.
In systems developed by Aerotech, for example, IFOV allows a single controller architecture for the laser scan head axes (Gx/Gy) as well as external linear axes to move the part (X/Y). The galvanometer servo drives, which control Gx/Gy, have physical external inputs for the encoder signals from the X/Y drives. All the drives connect using a fiber optic-based, low-latency communication bus; a real-time PC-based motion controller maintains control. IFOV further enables trajectories for the system to be performed with simple G-code commands sent to the laser scan head axes (Gx/Gy).
The IFOV works by generating commands for the X/Y servo axes based on the position commands sent to the scanner Gx/Gy axes. This is only possible because Gx/Gy axes operate at a trajectory rate of 200 kHz while the linear servo axes operate at 20 kHz — an order of magnitude higher. But all axes are synced together in real time.
This higher trajectory rate means that Gx/Gy can move ahead of X/Y, while X/Y executes its commands more slowly — allowing for more X/Y error without impacting the laser spot’s accuracy on
the printer bed. The laser’s positional
accuracy and speed are shared between the four axes of motion (Gx/Gy/X/Y), allowing for a constant processing speed across the entire travel path of the linear stages.
Laser spot placement accuracy
In addition to IFOV, position-based control of laser pulses further enhances the precision and efficiency of the 2PP process. Because a single laser pulse generates a voxel of cured material, inaccurate control over pulses will result in poor print quality. Position-synchronized output (PSO) is possible by synchronizing the pulse rate of a laser with the raw encoder feedback from the laser scan head axes (Gx/Gy). This ensures that laser pulses along the defined trajectory are spaced correctly and independently of how fast the scanner is moving for each layer.
PSO is a configurable tool that allows a user to define the spacing between pulses as well as the power level for each pulse. As the laser moves across a surface, the PSO trigger event becomes the current encoder-count position when that position meets a predefined event-type criterion. There is no software-based calculation or latency in this trigger event. The controller knows that the PSO output is driven directly by the associated axis position. This means that, whether the scanner speeds up or slows down, the spacing between laser pulses and their individual power level will deliver the exact process fluence. Ensuring a consistent fluence at every position further ensures consistently sized and accurately located voxels in a 2PP process.
PSO emerged as a solution for controlling laser triggering. It was developed because most laser processes require precise beam fluence and spot placement, but the high speed of laser scan head trajectories creates triggering challenges that inhibit such precision. PSO allows the scan
head to move rapidly across areas in which triggering is undesired, yet still maintain accurate placement of laser
spots without slowing down. Vector and raster-based laser trajectories can both benefit from this method of laser trigger control. The combination of PSO with IFOV allows both the laser scan head and linear axis encoders to trigger laser pulses.
Demonstrating the value
IFOV and PSO are more than engineering concepts. Both have demonstrated their practical capabilities in laser-based 3D microprocessing tools developed by
Femtika, a spinoff from the Laser
Research Center of Vilnius University
in Lithuania. The company’s tools are suitable for 2PP, laser ablation, and selective laser-etching processes.
Because a single laser pulse generates a voxel of cured material, inaccurate control over pulses will result in poor print quality.
The heart of their laser workstations always consists of a femtosecond laser in combination with a positioning system with nanometer accuracy. To achieve this accuracy, Femtika leverages linear stages and laser scan heads as well as IFOV and PSO controller technology developed by Aerotech.
When it first set out to develop these systems, Femtika worked under the
assumption that nanometer precision could only be achieved with piezo stages and a fixed optic, according to Vytautas
Purlys, Femtika’s CTO. The most common solution at the time was to mount a piezo stage on a larger, less precise mechanical stage.
Femtika found this approach sufficient
for processing small structures, but it
presented stitching problems and
extremely lengthy manufacturing when producing larger structures. Alternatively, using high-precision linear stages and galvanometers based on IFOV and PSO controller technology, Femtika saw both an improvement in quality when processing larger microstructures as well as an initial 10× increase in manufacturing speeds. Femtika found that it could increase processing speeds by a further factor of 10 by incorporating Aerotech’s laser scan head.
Where Femtika’s older piezo-based
2PP systems could produce a few dozen parts overnight, the use of the IFOV
and PSO features and advanced motion control hardware enabled it to produce thousands of high-quality structures within the same amount of time, Purlys said.
Improving the printing time by a factor of 20 enables the 2PP process to produce significantly larger parts with higher quality in less time. Further, it enables the micron-scale additive manufacturing process to become practical beyond the production of prototypes.
Looking forward
Advanced controller features such as IFOV and PSO directly address critical quality and throughput challenges for
2PP additive manufacturing processes as well as other laser microprocessing applications. It enables the cost-effective creation of highly repeatable parts at scale, which might just make a process that once seemed too slow or inaccurate to become a more practical and valuable manufacturing approach.
Acknowledgments
The authors wish to acknowledge Femtika’s experts in laser processing for their
contributions to and insights provided for
this article.